MATHEMATICAL, PHYSICAL AND CHEMICAL SCIENCES
UNDERLYING SANTILLI'S INTERMEDIATE NUCLEAR
SYNTHESES, WITHOUT RADIATIONS
Full scientific presentation available in the monograph
I. Gandzha and J Kadeisvili,
New Sciences for a New Era:
Mathematical, Physical and Chemical Discoveries of
Ruggero Maria Santilli, Sankata Printing Press, Nepal (2011),
http://www.santilli-foundation.org/docs/RMS.pdf
CHAPTER 7:
NUCLEAR ENERGIES WITHOUT RADIATIONS
7.1. INTRODUCTION
7.2. NEW HADRONIC ENERGIES OF ATOMIC TYPE
7.2A. Limitations of 20th century doctrines for energy releasing processes
7.2B. Conventional molecular combustion
7.2C. Santilli's magnecular combustion
7.3. NEW HADRONIC ENERGIES OF NUCLEAR TYPE
7.3A. Foreword
7.3B. Insufficiencies of "cold" and "hot" fusions
7.3C. Santilli's main idea for new controlled fusions
7.3D. Insufficiencies of quantum mechanics, quantum chemistry and special relativity for controlled fusions
7.3E. Insufficiencies of quark and neutrino conjectures for controlled nuclear fusions
7.3F. Basic assumptions of intermediate controlled nuclear fusions
7.3G. Physical laws of controlled nuclear fusions
7.3H. The role of Santilli magnecules for controlled nuclear fusions
7.3I. Engineering conception and realization of hadronic refineries
7.3J. The physics of intermediate controlled nuclear fusions
7.3K. Engineering conception of hadronic reactors
7.3L. Experimental verification of nitrogen synthesis without harmful radiations or waste
7.3M. Independent verification of Santilli's nitrogen synthesis without harmful radiation or waste
7.4. CLEAN ENERGY FROM THE STIMULATED DECAY OF THE NEUTRON.
7.4A. Introduction
7.4B. The stimulated decay of the neutron
<
7.4C. Neutron stimulated decay via photons with resonating frequency
7.4D. Hadronic energy of particle type
7.4E. Hadronic Energies via double beta decays
7.4F. Tsagas experiment on the Stimulated Neutron Decay
7.4G. Recycling of radioactive nuclear waste via their stimulated decay
7.5. THE STIMULATED DECAY OF RADIOACTIVE NUCLEAR WASTE
Under construction. Please visit the website
http://www.nuclearwasterecycling.com
7.6. CLEAN ENERGIES FROM THE NEW CLASS OF NUCLOIDS
,p>
To be uploaded. Visit in the meantime Section 6.4F of Chapter 6.
7.1. INTRODUCTION
Let us initiate the closing chapter of this book with the following statement released by Santilli:
Owing to increasingly alarming climatic events, whose violence is expected to increase in a progressively accelerating manner, the biggest need for the very survival of our society is the identification and industrial development of new clean energies. Consequently, the biggest duty of our scientific institutions, for which I see no comparison, is the discovery new, environmentally friendly energies.
A main problem is that, on one side, scientific institutions generally operate under a self-imposed mandate to conduct only the research which is in strict compliance with 20th century theories while, on the other side, all energies that could be conceived or are otherwise permitted by Einstein special relativity, quantum mechanics and quantum chemistry were fully identified by the middle of the past century and they all resulted as being environmentally unfriendly, either because of excessive production of green house gases, or because of the release of harmful radiation and/or radioactive nuclear waste nobody knows where or how to dispose of.
The dichotomy between our scientific institutions and the environmental need for mankind is rendered unreassuring, with a consequential variety of issues pertaining to scientific ethics and accountability, by the fact that Einstein special relativity, quantum mechanics and quantum chemistry are strictly reversible over time, while all energy releasing processes are strictly irreversible. Hence the belief that the former preferred doctrines are exactly valid for the latter processes is amoral, asocial and ascientific, since the only possible debatable issue is the selection of the appropriate generalizations-coverings.
When faced with the above evidence, often studiously ignored, a traditional objection that is voiced with rapidly propagating support is that the irreversibility of our physical reality is only "apparent" (sic) because, so the claim states, when the irreversible macroscopic event is reduced to its elementary particles constituents, the irreversibility "disappears" (sic) and one recovers conventional theories.
However, I proved decades ago the following property that has been also propagated in the physics community but equally ignored, thus without disproof:
THEOREM: A macroscopic process that is irreversible over time cannot be consistently decomposed into a finite number of elementary particles all reversible over time and, vice versa, a finite number of elementary particles all reversible over time cannot yield a macroscopic irreversible process under the correspondence or any other principle.
The implication of the above theorem, whose proof has been confirmed by first year graduate students, are rather deep. It implies that, rather than "disappearing" to allow the applicability of a preferred theory, the origin of irreversibility rests at the most ultimate level of nature, that of elementary particles. As an example, the irreversibility of a spaceship during reentry in our atmosphere originates from nonlinear, nonlocal an nonpotential interactions between the electron orbitals of peripheral atoms of the spaceship and the corresponding orbitals of atmospheric atoms.
In view of the above scenario, the only serious hope for mankind to resolve our alarming environmental problems is to build, test and establish irreversible generalizations-coverings of Einstein special relativity, quantum mechanics and quantum chemistry specifically conceived for the conception, testing and industrial development of new clean energies.
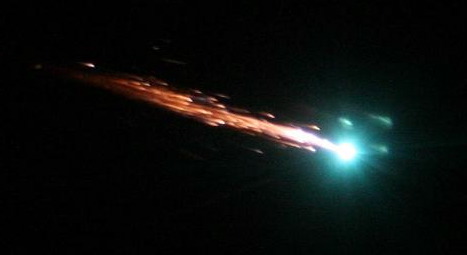
Figure 7.1. A NASA picture of a spaceship during reentry in our atmosphere often used by Santilli to illustrate visual experimental evidence often ignored by academia, the irreversibility of interior dynamical events, the impossibility of their serious reduction to elementary particles in nice reversible conditions, and the consequential need to achieve, in due time, an irreversible covering of the entire 20th century sciences.
To my best knowledge, the Lie-isotopic and Lie-admissible relativity, and related branches of hadronic mechanics and chemistry, are the only broadening of conventional doctrines verifying the conditions: 1) Directly universality for the representation of interior dynamical systems of extended particles within physical media; 2) Invariance under their own time evolutions so as to avoid catastrophic inconsistencies; and 3) Unique and unambiguous admission of conventional doctrines as simple particular cases whenever exterior conditions of point particles in vacuum are recovered. In particular, the new covering disciplines and their novel underlying mathematics have achieved operational maturity, as proved by experimental verifications in all quantitative sciences, as well as the prediction and industrial development of new clean energies.
In this chapter, we review the new clean energies permitted by Santilli Lie-isotopic and Lie-admissible classical and operational formulations in atomic physics, nuclear physics and particle physics. A main objective of the presentation is to show that the new energies studied in this chapter are prohibited by 20th century doctrines and, consequently, they are crucially dependent on "deviations" from orthodox theories.
The main book underlying this chapter is given by the work (hereon referred to as the 1999 monograph):
The Physics of New Clean Energies and Fuels According to
Hadronic Mechanics,
R.M. Santilli,
Special issue of the Journal of New Energy, 318 pages (1999).
The second main reference of this chapter is Santilli's subsequent work (hereon referred to as the 2001 monograph)
"Foundations of Hadronic Chemistry,
with Applications to New Clean Energies and Fuels"
R. M. Santilli,
Kluwer Academic Publishers (2001)
A comprehensive treatment of the new energies is presented in Santilli's five volumes (hereon denoted 2008 monographs)
Hadronic Mathematics, Mechanics and Chemistry, Volumes I, II, III, IV and V,
R. M. Santilli,
International Academic Press (2008)
Additional papers will be quoted later on when needed. An in depth knowledge of hadronic mechanics and chemistry as outlined in Chapters 3 and 4, respectively, is necessary for a technical understanding of this chapter, let alone to prevent the illusion of serious criticisms.
7.2. INAPPLICABILITY OPF 20TH CENTURY METHODS FOR ENERGY RELEASING PROCESSES
In this section, we review a new form of energy based on a new form of novel combustion, today known as Santilli magnecular combustion, permitted by hadronic mechanics and chemistry whose very existence is crucially dependent on deviations from 20th century chemistry. For technical details one may consult Santilli 2001 and 2008 monographs.
One of the most serious constrain in the evolution of science is the belief, rather widespread in the scientific community, that Einstein special relativity, quantum mechanics and quantum chemistry apply for all conceivable conditions existing in the universe, expectedly until the end of time. Unreassuringly such a belief is the very reason for the lack of resolution until now of our alarming environmental problems since said belief restrains the conception, let alone development of new clean energies.
In the preceding chapters, we have reviewed rather vast evidence on the limitations of 20th century theories in all quantitative sciences and their resolution by Santilli Lie-isotopic and/or Lie-admissible formulations. In preparation for the content of this section, we recall that Maxwell equations, the Lorentz-Poincare' symmetry and Einstein special relativity describe quite well an electric arc in vacuum (see Figure 4.16). However, when dealing with corresponding interior conditions, such as an electric arc in water, Santilli has identified a number of basic insufficiencies all with damaging environmental implications.
To begin, Santilli indicates that there is no need to conduct measurements for admitting the inapplicability of 20th century doctrines for a submerged electric arc because of the evident and well known facts that the very basic notions of electric resistance, entropy and other thermodynamical laws crucial for interior dynamical problems are irreconcilably incompatible with Einstein special relativity, quantum mechanics and quantum chemistry. It is evident that, without the capability of defining resistance, entropy and thermodynamical laws ab initio from primitive axioms, any description of submerged electric arc with 20th century theories is flawed at best, or denoting theological preferences.
In addition to the above basic evidence, Santilli has indeed conducted comprehensive measurements and established the following deviations of experiential evidence on submerged electric arcs from Einstein special relativity, quantum mechanics and quantum, chemistry that are so large to prevent even the usual use of unknown functions thrown into the equations "to adjust things" details (see the website www.magnegas.com):
1) Quantum mechanics and chemistry predict that a DC arc between carbon electrodes submerged within distilled water produces a combustible gas composed by about 65% H2, 32% CO and 2% to 3% H2O, CO2 and other molecules. But CO is combustible in air producing CO2. Hence, quantum mechanics and chemistry predict that the combustion exhaust of said gas should contain about 40% CO2. Numerous measurements have established that the combustion exhaust of said gas contains about 4% to 6% of CO2 thus establishing close to a ten-fold deviation of the predictions of quantum mechanics and chemistry from measured data. Since CO2 is the gas responsible for increasingly alarming climactic events, the study of combustible gases produced by submerged electric arcs cannot be solely done with 20th century doctrines without raising issues pertaining to scientific ethics and accountability.
2) Quantum mechanics and chemistry predict that the combustion exhaust of the above gas in an exact stochyometric ratio with oxygen does not contain appreciable percentage of O2, said exhaust being composed by H2O, CO2 and small impurities. On the contrary, various measurements have established that the indicated combustion exhaust contains up to 14% breathable oxygen, thus establishing another large deviation of 20th century doctrines from reality. Since oxygen is the very essence of life, the insistence in the study of the indicated gas via 20th century doctrines is clearly not warranted for the solution of environmental problems.
3) By remembering that H2 contains about 300 BTU/scf and CO about 89 BTU/scf, according to quantum mechanics and chemistry the above combustible gas contains about 240 BTU/scf. However, clear experimental evidence establishes that said gas cuts 5" metal plates faster than acetylene that contains 2,400 BTU/scf, thus establishing yet another large deviation of 20th century theories from experimental evidence.
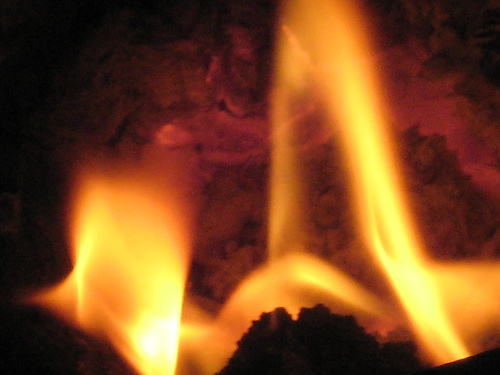
Figure 7.2. In Santilli's view, tens of thousand of years have passed since mankind discovered fire, but we are still far from achieving a quantitative representation of combustion at the primitive level of valence electron bonds for numerous reasons, such as: the irreversible character of combustion compared to the reversible character of quantum mechanics and chemistry; the absence in 20th century chemistry of a quantitative identification of the attractive force in a valence bond; and other insufficiencies discussed in Chapter 4.
The above occurrences prove beyond scientific doubt that a quantitative understanding of the deviations from Einstein special relativity, quantum mechanics and quantum chemistry has primary relevance for the solution of our environmental problems.
7.3. NEW HADRONIC ENERGIES OF NUCLEAR TYPE
7.3A. Foreword
In this section, we outline new clean energies that can be predicted and developed at the nuclear level via the use of hadronic mechanics, for which reason they are called new hadronic energies of nuclear type.
The main aspect is that all nuclear energies that could be conceived via the use of quantum mechanics were fully known by the middle of the 20th century and they all turned out as being environmentally unacceptable because of the production of harmful radiations, such as those composed by penetrating neutrons fluxes, and they released radioactive nuclear waste our society has been unable to dispose of in an environmentally acceptable way.
Santilli has: 1) studied existing nuclear energies for decades; 2) identified the insufficiencies of quantum mechanics in nuclear physics; 3) constructed a covering of quantum mechanics specifically conceived for nuclear structures; 4) predicted new form of nuclear energies without harmful radiations or radioactive waste that are inconceivable for quantum mechanics, but are predicted by the covering hadronic mechanics; 5) achieved their experimental verification and solicited their independent experimental confirmation.
Following over thirty years of preparatory mathematical and physical research, Santilli presented the first type of new, clean, hadronic energies of nuclear type in the 1999 and 2001 monographs and then in the 2008 memoir
The novel "Intermediate Controlled Nuclear Fusion:" a report on its industrial realization as predicted by hadronic mechanics.
R. M. Santilli
Hadronic Journal 31, pages 115-200 (2008),
The experimental verification of the new energy with particular reference to the absence of neutron or other harmful radiations was achieved in the 2010 paper
Experimental confirmation of the novel "Intermediate Controlled Nuclear Fusion" without harmful radiations
R. M. Santilli
New Advances in Physics, in press (2010),
The independent experimental verification of the new energy with particular reference, again, to the absence of harmful radiations, was done also in 2010 by three nuclear physicists from a company in Princeton, New Jersey, and it is available in the paper
Verification of Santilli's intermediate nuclear fusions without harmful radiation
and the production of magnecular clusters
R. Brenna, T. Kuliczkowski, L. Ying
New Advances in Physics, in press (2010),
A conceptual - nontechnical outline of the new energy is given by the paper
Outline of the novel intermediate controlled nuclear fusions
R. Anderson,
English version
Russian version
Italian version
Turkish version
French version
7.3B. Insufficiencies of "cold" and "hot" fusions
Following the pioneering research by Fleishmann, Pons and Hawkins of 1989, vast
research has been conducted on Low Energy Nuclear Syntheses (LENS) popularly called ''Cold Fusion" (CF), reviewed in a large variety of publications not quoted here to avoid discriminatory listing.
Despite vast research, the field has remained controversial because of a rather widespread view that there is no sufficient evidence to conclude at this writing that LENS do or do not occur due to claimed insufficient precision of available calorimeters, or the claimed lack of consistent reproduction of the results.
Additional reasons for the controversies are: claimed incompatibilities of LENS with pre-existing theories, despite the availability of new theories; denial of "cold fusions" on grounds of lack of emission of neutrons, while fusions without any emission of neutrons are possible; and other arguments.
Following extensive theoretical, experimental and industrial research in the field, Santilli's view is that LENS have indeed occurred in numerous tests, although the syntheses occurred at random, thus without hope of achieving industrial relevance, namely, the production of an energy surplus suitable to justify the construction of new electric power plants based on LENS. Recent additional experiments have confirmed the occurrence of LENS in laboratory, but the energy surplus necessary for industrial relevance has remained vastly elusive.
Additional research supported by a collective international investment
of about one billion dollars over the past fifty years has shown that the High Energy Nuclear Syntheses (HENS), popularly called ''Hot Fusion'' (HF), can indeed be attained in laboratory, although the latter fusion too has not achieved industrial significance, and none is in sight at this writing, due to uncontrollable instabilities at the initiation of the fusion process, and other reasons.
In view of the decades of inability to achieve results of clear industrial value despite the expenditure of vast public and private funds, Santilli re-examined the foundations of fusion research, beginning with a re-examination of the basic theories used for all nuclear fusions, particularly in references to quantum mechanics, quantum chemistry and special relativity.
By keeping in mind the need by our society for new clean energies, and the fact that said disciplines were conceived and verified for conditions dramatically different than nuclear fusions, it is questionable to assume aprioristically and without serious scrutiny the exact validity of 20th century disciplines for fusion processes.
As recalled earlier, quantum mechanics, quantum chemistry and special relativity were conceived, constructed and verified for reversible processes, namely, processes whose time reversal images are causal, such as electron orbits in atomic structures, particles moving in accelerators, and many other systems. By contrast, all nuclear synthesis constitute strictly irreversible processes, namely, processes whose time reversal images violate causality and other laws.
As experts are expected to know for qualifying as such, the quantum mechanical probability amplitude for a Hermitean Hamiltonian is time reversal invariant. Consequently, quantum mechanics does predict a finite probability for two nuclei N1, N2, to fuse into a third nucleus N3 with the consequential release of energy ΔE given by the difference between the initial and final rest energies
(7.9) N1 + N2 → N3 + ΔE,
(7.10) ΔE = E3 - (E1 + E2).
However, in view of said time reversal invariance, quantum mechanics also predicts a finite probability for the spontaneous time reversal decomposition of the third nucleus into the original two,
(7.11) N3 → N1 + N2,
in gross violation of the principle of conservation of the energy, causality, and other basic laws.
Consequently, any posture that strictly reversible theories, such as quantum mechanics, quantum chemistry and special relativity, are exactly valid for irreversible processes such as nuclear fusions, is ascientific, because the selection of irreversible covering theories more appropriate for the description of nuclear fusions is indeed open to scientific debate, but not their need.
7.3C. Santilli's main idea for new controlled fusions
In view of the above protracted insufficiencies at low and high energies, in his 2008 paper Santilli proposed, apparently for the first time, a new type of nuclear synthesis under the name of Intermediate Controlled Nuclear Fusions (ICNF), or ''Intermediate Fusion'' (IF) for short.
Recall that atoms are normally protected by their electron clouds and that nuclei have to be systematically exposed out of such clouds for their fusion to occur in a systematic fashion. A main shortcoming of the ''cold fusion'' is that the available energy is generally insufficient to control atomic electron clouds so as to expose nuclei, in which case no fusion is systematically possible.
For the case of the ''hot fusion,'' we have the opposite occurrence in which atoms are completely stripped out of their electron clouds, but the energies are simply excessive, thus preventing the possibility of a real control of nuclear fusions, as well known in particle scattering processes in which excessive collision energies prevent absorption.
The name ''intermediate'' was proposed by Santilli to denote that the available energy is indeed intermediate between those of the ''cold'' and ''hot'' fusions, thus avoiding the shortcomings of both fusions. More particularly, the available energy for the proposed intermediate fusion is set to a namely the minimal value of energy sufficient for the control of atomic clouds to expose nuclei, verify all conservation laws and control their synthesis.
The above conditions are verified, e.g., for the plasma created by an electric arc, which plasma is typically at about 10,000o F, thus having an energy that cannot be qualified as belonging to either the '"cold" or the ''hot'' fusion. The energy is then carefully selected to have a additional minimal value for fusions to occur, so as to avoid the indicated impossibility for controlled fusions under excessive collision energies. Priority is then given to the identification of the physical laws to be verified for systematic, industrially viable fusions and their engineering realizations.
It should be indicated that numerous plasmas have been used in ''cold fusion'' research. Nevertheless, dramatic differences will soon emerge between Santilli's intermediate fusion and existing plasma fusion research, due to irreconcilable differences in the assumed basic laws. Santilli then proposed in his 1999 and 2001 monographs as well as in his 2008 specific reactors, called hadronic reactors because based on hadronic mechanics and chemistry, for the possible
industrial utilization of the clean energy expected from his intermediate fusions.
To achieve this task, Santilli: 1) Identified the basic disciplines that are applicable to all controlled fusions, whether ''cold,'' ''intermediate'' or ''hot''; 2) Identified the
basic laws that have to be verified for any controlled fusion to occur; and 3) Proposed in
manufacturing details specific hadronic reactors based on the realization and optimization of said physical laws.
7.3D. Insufficiencies of quantum mechanics, quantum chemistry and special relativity for controlled fusions
In his 2008 memoir, Santilli confirmed his preceding studies on the lack of exact character of 20th century theories for nuclear fusions, and pointed out that basically new, potentially clean energies are expected to be predictable by deviations from said theories, no matter how small. These insufficiencies have been outlined in Chapter 1 of this volume and studied in details in the subsequent chapters. Therefore, they will be considered as being known hereon.
It should be indicated that Santilli moves no main objection in the use of quantum mechanics for nuclear fissions because, in this case, the debris of the fission can be effectively represented as being massive points moving in the inter atomic vacuum, thus allowing a serious applicability of the mathematical and physical foundations of quantum mechanics. Santilli moves objections against the assumption of quantum mechanics as being exactly valid for nuclear fusions because, unlike the case of fissions, nuclei cannot any longer be credibly approximated as being massive points since they must be fused together.
As a matter of fact, we shall show below that the inability of "cold fusion" to achieve industrial relevance is mostly due to the excessive approximation of nuclei as massive point, an approximation absolutely necessary for the applicability of the foundations of quantum mechanics, because said approximation causes the violation of basic physical laws for all fusions, such as the proper spin couplings of extended nuclei, which coupling become unnecessary under point-like abstractions.
Among the various formulations of hadronic mechanics specifically applicable to nuclear physics, we shall tacitly use in the following the memoir for the Lie-isotopic case, namely, for the structure of stable nuclei:
Nuclear realization of hadronic mechanics
and the exact representation of nuclear magnetic moments
R. M. Santilli,
Intern. J. of Phys. Vol. 4, 1-70 (1998)
and the additional memoir for the broader irreversible case, namely, for the study of unstable nuclei and nuclear fusions:
Lie-admissible invariant representation of irreversibility for matter and antimatter at the classical and operator level
Ruggero Maria Santilli
Nuovo Cimento B Vol. 121, p. 443-595 (2006)
7.3E. Insufficiencies of quark and neutrino conjectures for controlled nuclear fusions
In his 2008 memoir, Santilli reviews the negative implications, particularly for the conception of new clean nuclear energies, caused by the indiscriminate assumption of quarks and neutrinos as being physical particles existing in our spacetime without a serious scrutiny.
As reviewed earlier, Santilli accepts as final the Mendeleev-type classification of hadrons into families achieved by SU(3)-color models, confirms the need of quarks for their elaboration, but assumes that quarks are what they technically are: purely mathematical entities characterized by mathematical representation of a purely mathematical symmetry defined on a purely mathematical, complex-valued internal space that, as such, dismiss any hope of quantitative definition in our spacetime. In any case, Santilli recalls the impossibility for quarks to have gravity as conceived by Einstein, and other basic shortcomings reviewed earlier.
The 2008 memoir then points out the negative implications for the conception of new energies whenever protons and neutrons, thus nuclei, are assumed as being composite systems of quarks as physical particles in our spacetime. These implications will perhaps appear more forcefully in the next section when studying the new energies originating in the structure of individual hadrons, rather than in their collection.
The 2008 memoir then suggests caution in the additional assumption of the plethora of neutrinos predicted by the standard model as being physical particles in our spacetime. In this respect, Santilli first recalls the basic insufficiency of the historical Pauli-Fermi hypothesis for a quantitative representation of the first and most fundamental synthesis in nature, that of the neutron from a hydrogen gas studied in details in the preceding chapter.
Santilli then illustrates the insufficiencies for the plethora of hypothetical, massive and oscillating neutrinos predicted by the standard model and the dependence of any model of clear fusion from neutrino conjecture whenever the latter are assumed as physical particles in our spacetime. The evident argument is that, once the insufficiency of the hysterical Pauli-Fermi hypothesis of the neutrino are technically identified for the fundamental synthesis of the neutron, the corresponding insufficiencies for all subsequent nuclear fusions become transparent and compelling.
In view of the above, Santilli certainly does not object on the continuation of research on nuclear fusions based on the assumption of quarks and neutrinos as physical particles, but strongly recommend the joint research without the assumption of hypothetical particles and the use instead of covering theories, since the comparison of the two approaches clearly shows the capability by the latter of predicting new clean energies that are prohibited for the former.
With regard to the above aspects, a pre-requisite for the technical understanding of the ICNF and their industrial development is a study of the paper
The etherino and/or the neutrino hypothesis
R. M. Santilli,
Foundation of Physics, Vol. 37, 670 (2007)
7.3F. Basic assumptions of intermediate controlled nuclear fusions
Following the preceding studies, in his 2008 memoir, Santilli makes the following basic assumptions for his ICNF:
1) Nuclear force. Virtually the entire 20th century research in nuclear physics, thus including research in "cold" and "hot" fusions, has been based on the assumption that the nuclear force is entirely derivable from a potential. Consequently, all nuclear structures and their processes were entirely represented with a Hamiltonian, a condition evidently necessary for the applicability of quantum mechanics. By contrast, Santilli central assumption is that part of the nuclear force is indeed of action-at-a-distance, potential type representable with a Hamiltonian, and part is of contact, nonpotential type that cannot be represented with a Hamiltonian. This central assumption implies that the time evolution of nuclear structure and processes is necessarily of nonunitary type. The use of the various branches of hadronic mechanics then emerges as the only known axiomatically consistent and time invariant nonunitary formulations of nuclear structures and their processes.
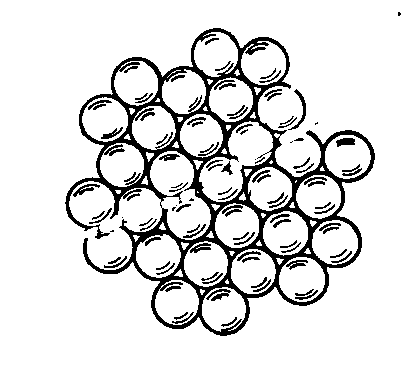
Figure 7.5. A reproduction of Figure 6.24 often used by Santilli to illustrate that "nuclei have no nuclei" being composed by extended constituents in contact with each other (technically, in conditions of mutual penetration of about 10-3 of their
charge distributions). Consequently, the nuclear force is expected to be partially of potential and partially of nonpotential type, with ensuing nonunitary character of the theory, and related applicability of hadronic mechanics.
2) Stable nuclei. In this case, Santilli assumes the Lie-isotopic branch of hadronic mechanics based on the dynamical equations he proposed in 1978 when a member of Harvard University for stable, reversible, interior dynamical problems, today known as Heisenberg-Santilli Lie-isotopic equations here recalled for the time evolution of a Hermitean operator A in the infinitesimal and finite forms (see Section 3,11 for detailed treatments and references)
(7.12a) i dA/dt = [A, H]* = ATH - HTA,
(7.12b) A(t) = exp(HTti) A(0) exp(-itTH),
in which: the Hermitean Hamiltonian
(7.13) H = p2/2m + V(r)
represents all possible nuclear forces truly derivable from a potential V(r); the isotopic element T represents all contact nonpotential interactions allowing a nuclear structure with all constituents in actual contact of each other, and simplest possible realizations of the type
(7.14) T = exp [ - F(r) ∫ ψ†(r) ψ(r) d3r] > 0,
recovering quantum mechanics identically and uniquely when there is no appreciable overlapping of the wavefunctions ψ of nuclear constituents; and the inverse of the isotopic element,
(7.15) I* = 1/T > 0,
represents the basic, right and left unit of the theory at all levels, including numbers, differential calculus, functional analysis, etc. The presence of contact, non-Hamiltonian interactions is then assured by nontrivial values of T. The stability of the nucleus (reversibility over time) is represented by the identity of the basic isounit to the right and to the left, namely, for motions forward and backward in time.
3) Unstable nuclei and nuclear fusions. In this case, Santilli assumes as the main discipline the Lie-admissible branch of hadronic mechanics with basic dynamical equations he also proposed in 1978 and today known as Heisenberg-Santilli Lie-admissible equations here presented for the time evolution of a Hermitean operator A also in their infinitesimal and finite forms
(7.16a) i dA/dt = (A, H)* = ARH - HSA,
(7.16b) A(t) = exp(HSti) A(0) exp(-itRH),
where H continues to be Hermitean although it now represent the nonconserved total energy, and the nonpotential interactions are represented by the genotopic elements R and S. Irreversibility is assured in this case by the different values of the genounit for forward (fw) and backward (bw) motions in time,
(7.17) Ifw = 1/R ≠ bwI = 1/S..
In this case, the Lie-admissible branch of hadronic mechanics is ideally suited to represent the decay of unstable nuclei as well as nuclear fusions, since both are irreversible over time.
4) Neutron synthesis. As recalled earlier, stars initiate their lives as being composed of hydrogen; then they first synthesize the neutron from the compressed hydrogen gas; and then they synthesize all natural elements. Consequently, the synthesis of the neutron is the first and most fundamental nuclear synthesis. For this reason, Santilli spent decades of his research life for a quantitative understanding of the synthesis of the neutron from a proton and an electron, resulting in the reaction studied in details in the preceding chapter
(7.18) p+ + a + e- → n,
where "a" represents Santilli's etherino which is not intended as being a physical particle, but rather to represent in a conventional Hilbert space the transfer of 0.782 MeV and spin 1/2 missing in the synthesis of the neutron from the environment to the neutron structure.
To understand the new hadronic energies, the reader should keep in mind the impossibility of using the Pauli-Fermi neutrino conjecture for the synthesis of the neutron due to the inconsistency of the conventional Schroedinger's equation under the positive potential (see the preceding chapter for details).
To reach a more technical understanding of the new energies, the reader should also know that the etherino disappears at the covering level of hadronic mechanics and the formulation of the neutron synthesis on a iso-Hilbert space over an isofields.
Finally, the reader should meditate a moment on the origin of the missing 0.782 MeV for the synthesis of the neutron because, in the event such energy is provided by the environment, a star would never start producing light due to enormous energy needed for the synthesis of an enormous number of neutrons, This lead Santilli to a return to a continuous creation in our universe with the missing energy provided by the ether as a universal substratum (see the preceding chapter for details).
5) Nuclear structure. Rather than attempting to achieve the joint classification of baryons into a family and the structure of each members of the baryonic family via three hypothetical quarks, Santilli assumes the unitary classification of baryons as valid, but introduces new structure models of each member of the baryonic family with physical constituents, namely, constituents that can be produced free thus being detected by our instruments in our spacetime. Resolution of historical objections is merely achieved by assuming that, when in interior conditions (only), barionic constituents obey covering mechanics and symmetries with ensuing mutations (denoted with an asterisk) of their intrinsic characteristics. In particular, Santilli assumes the proton as an elementary stable particle without known structure (in view of its stability), and assumes the neutron as an unstable particle composed by one proton p*+ and an electron e*- in mutated conditions due to their total mutual immersion and resulting synthesis
(7.19) n = (p*+, e*-)hm,
as described in details in Section 6.3, hereon assumed as known.
Consequently, Santilli assumes that nuclei are indeed a collection of protons and neutrons, but only in first approximation, while being at a deeper level a collection of mutated protons and mutated electrons. As we shall see, the latter assumption alone allows far reaching studies on new clean energies.
7.3G. Physical laws of controlled nuclear fusions without radiations
One of the first contributions of hadronic mechanics, hadronic chemistry and isorelativity to new clean nuclear energies is the identification, apparently for the first time, of basic physical laws that have to be obeyed by all nuclear fusions to occur in a systematic (rather than in a random) way. Such laws must then be subject to engineering realization and optimization for nuclear fusions to acquire industrial value. Said laws apply for all fusions, thus for "cold," "intermediate" and "hot" fusions, they were first published in the 1999 monograph, are referred to in the literature as Santilli's laws for controlled nuclear fusions Let us begin with the following:
DEFINITION 7.3G: Controlled Nuclear Fusions (CNF) are given by systematic energy releasing nuclear fusions whose rate of synthesis (or of energy output) is controllable via one or more mechanisms capable of performing the engineering optimization of the applicable laws.
We are not in a position to review here the derivation of the basic laws of CNF to avoid a prohibitive length and we merely provide their conceptual outline with a few comments (for a more detailed treatment one may study the 2008 memoir).
LAW I: A necessary condition for CNF to occur is to control the orbitals of peripheral atomic
electrons in such a way to allow nuclei to be systematically exposed. Nature has set matter in
such a way that nuclei are shielded by their atomic clouds. It is evident that a
''nuclear'' synthesis between two conventional ''atoms'' is impossible at low energies
because the electron clouds will never allow nuclei to approach each other, let alone
to synthesize a new nucleus. This law explains the inability of the ''cold" fusions to
achieve industrial significance in energy output because, by definition, ''cold fusions'' do not
have the energy necessary for the ionization of atoms. This law also illustrates the need for the proposed ''intermediate fusions'' in which the first energy requirement is precisely the control of atomic clouds.
LAW II: CNF only occur among nuclei whose spins are either in ''singlet planar
coupling'' or ''triplet axial coupling" (see Figure 7.6). This
law illustrates the structural differences between quantum and hadronic mechanics,
as well as the necessity of the latter for CNF to occur systematically. The constituents of a bound state of two quantum particles must necessarily be point-like to avoid structural
inconsistencies beginning with the local-differential topology.
Consequently, singlet and triplet couplings are equally possible for quantum mechanics. When the actual extended character of the constituents is taken into account, it is easy to see that triplet planar couplings of extended particles at short distances are strongly repulsive, while singlet planar couplings are strongly attractive, where the word ''planar'' is intended to indicate that the two nuclei have a common median plane, while "axial" indicates a common axial symmetry (Figure 7.6). This law was introduced by Santilli in the original 1978 proposal to build hadronic mechanics via the so-called gear model. In fact, the coupling of gears in triplet (parallel spins) causes extreme repulsion, while the only possible coupling of gears is in singlet (antiparallel spins). The emergence of a strongly attractive force for the singlet planar or triplet axial couplings is one of the fundamental contributions of hadronic mechanics to fusion processes since such a force is totally absent for quantum mechanics, while it appears naturally in all spinning and deeply overlapping particles, as established in the Cooper pair in superconductivity, the valence bonds in chemistry, the synthesis of neutrons from protons and electrons, the strong nuclear binding energy, and other cases.
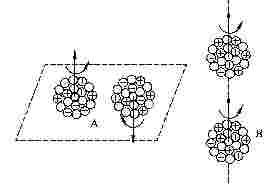
Figure 7.6. A schematic view of the only two stable couplings permitted by hadronic mechanics for nuclear fusions, the ''singlet planar coupling'' of the l.h.s. and the ''triplet axial coupling'' of the r.h.s. All other spin configurations have been proved to produce strongly ''repulsive'' forces under which no CNF is systematically possible.
LAW III: The most probable CNF are those occurring at threshold energies (namely, at
the minimum value of the energy of the original nuclei needed to verify all laws). This hadronic law evidently identifies the main reason for the proposed "intermediate nuclear fusions." A main reason of the law is that all energies below said threshold value do not allow industrially meaningful nuclear syntheses (this is the case for "cold" fusions), and all energies above the indicated threshold value cause instability that reduce the rate of synthesis in a way proportional to the energy excess (this is the case for "hot" fusions). As we shall see, the lack of
engineering implementation of this law constitutes another reason ''cold'' and ''hot'' fusions
have not achieved industrial relevance until now.
LAW IV: The most probable CNF are those without the release of massive particles
(such as protons, neutrons and alpha particles). This law was not expected by Santilli. Yet,
contrary to popular beliefs, explicit calculations based on hadronic mechanics indicated that the probability of a nuclear synthesis with the
release of neutrons is much smaller than that of another synthesis without the emission
of massive particles. This law has been verified by ICNF achieved to date and, apparently, this law appears to be verified by nuclear syntheses
spontaneously occurring in nature. It should be stressed that this law does not preclude the study of CNF with secondary emission of massive particles. It only suggests the preferred study of nuclear syntheses without release of massive particles for evident environmental reasons.
LAW V: CNF cannot occur without a "trigger," referred to an external mechanism forcing exposed nuclei to pass through the hadronic horizon. All nuclei are positively charged, thus repelling each other at distances bigger than one Fermi. Without a mechanism that overcomes the Coulomb repulsion and brings nuclei inside the hadronic horizon, no nuclear synthesis is evidently possible. By contrast, when inside the hadronic horizon and the preceding laws are verified (with particular reference to law II on spin couplings), the synthesis is inevitable due to the activation of the strongly attractive hadronic forces that overcome the repulsive Coulomb force. The case is similar to that of identical valence electrons that evidently repel each other for all distances bigger than one Fermi (range of validity of quantum mechanics and potential interactions), but strongly attract each other at mutual distances less than one Fermi (range of validity of hadronic mechanics and nonpotential interactions).
It is instructive to examine a representative case of ''cold" fusion under the above physical
laws. Consider the Fleishmann-Pons electrolytic cell.. It is easy to see that
this cell does indeed verify the conservation of the energy, the conservation of the
angular momentum, and admits a trigger characterized by the electrostatic pressure compressing deuterium inside the palladium.
However, Fleishmann-Pons electrolytic cell does not verify Laws I (control of atomic clouds to expose nuclei), Law II (control of spin couplings) and other laws . In fact, nuclear
spin couplings occur at random, there is no clearly identified mechanism to expose nuclei, and there is an equally clear lack of optimization of the verified laws. Consequently, nuclear syntheses occur at random, thus preventing industrial values of the energy output.
It is an instructive exercise to inspect other realizations of ''cold" fusions" among the large variety existing in the easily identifiable literature (not quoted here to avoid discriminatory listings). One can see in this way that, to our best knowledge at this time, none of available ''cold" fusions" realizes ''all'' basic hadronic laws (the indication of the contrary would be appreciated).
7.3H. The role of Santilli magnecules for controlled nuclear fusions
Inspection of CNF Laws I-V reveals that the most difficult engineering realization is that of Law I on the systematic control of electron clouds to expose
nuclei as a pre-requisite for their fusion. Following a decade of research, and thanks to large private funds, Santilli achieved industrial maturity for gaseous and liquid fuels via the new chemical species of magnecules studied in details in Chapter 4, currently sold in various countries by a number of corporations (see www.magnegas.com).
As illustrated in Figure 7.7, Santilli magnecules do indeed achieve the desired systematic and controlled exposure of nuclei in such a way to be naturally set to verify Law II on the spin coupling. In particular, said coupling is of axial triplet type, namely, the most efficient for nuclear fusions to our knowledge.
Rather remarkably, Santilli has indicated various times in his writings that the new chemical species of magnecules was conceived as a necessary preparatory step for the achievement of his intermediate controlled fusion. Inspection of Figure 7.7 indicates that, when atoms are reduced to toroids and aligned in axial triplet coupling, nuclear fusion merely require an external action, the trigger, to push nuclei at one Fermi mutual distance at which point the strongly attractive nuclear force is activate and fusion becomes inevitable.
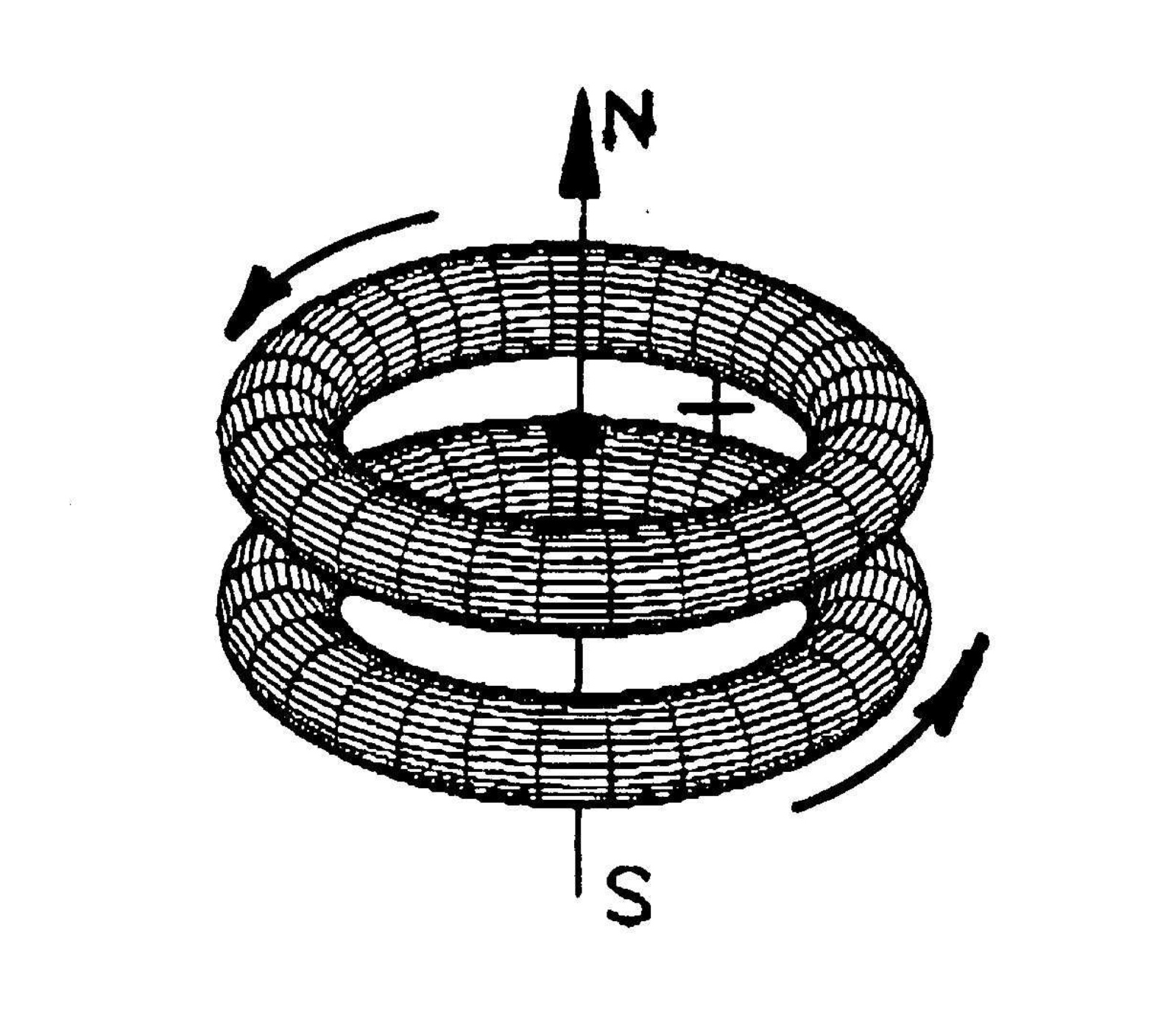
Figure 7.7. A reproduction of Figure 4.11 to illustrate the fundamental role of Santilli magnecules for controlled nuclear fusion since they assure the correct spin coupling as per Law II.
For notational purposes, we recall that conventional valence bonds are indicated with the symbol "-", while Santilli magnecular bonds are indicated with the symbol "x". Hence, the hydrogen molecule is indicated with the usual symbol
(7.20) H2 = H - H,
while magnecular hydrogen, denoted with the symbol MHn is written (see Chapter 4 for more details)
(7.21) MH2 = HxH, MH3 = HxHxH or (H-H)xH, etc..
It is possible to see that, under suitable engineering optimization studied in the next sections, the magnecular structure of Figure 7.7 also allows the verification of the remaining laws of controlled nuclear fusions.
7.3I. Engineering conception and realization of hadronic refineries
Santilli has conceived and constructed the the following two types of equipment:
1) Hadronic Refineries denoting an equipment suitable for the recycling of liquid wastes into a gaseous fuel with magnecular structure (called magnegases) via a submerged electric arc; and
2) Hadronic Reactors, denoting an equipment primarily devoted for the production of industrially significant heat via an electric arc submerged within a properly selected fluid.
Both reactors have the name "hadronic" because based on a submerged electric arc that, as such, requires the use of hadronic mechanics and chemistry due to excessive deviations from quantum mechanics (see Chapters 1 and 4).
In this section, we outline engineering details on hadronic refineries, and pass later on to the more complex hadronic reactors of main interest for this chapter. The waste used in refineries is called liquid feedstock, while the gas used in reactors is called hadronic fuel.
One of the biggest engineering difficulties for the industrial production of the new chemical species of Santilli magnecules, whether for hadronic refineries or reactors, is that the toroidal polarization of spherical atomic orbitals requires very strong magnetic fields of the order of billions of Gauss, which fields have to be realized in an industrially viable and cost effective way (for details, see Appendix 8A of the 2001 monograph). The solution identified by Santilli following long studies is that via the use of DC electric arcs submerged within a properly selected liquid feedstock.
With reference to Figure 7.8, at atomic distances from the DC arc with 100 kW power, the magnetic field can reach values of 1011 Gauss or more, thus being sufficient for the desired polarization of atomic orbitals. Additionally, said magnetic field naturally couples polarized atoms into magnecules with triplet axial coupling North-South-North-South- .... Finally, the arc compresses magnecules toward its axial symmetry at the time of its disconnect and re-initiation (for reasons not entirely understood so far), thus favoring the realization of the "trigger" for the systematic achievement of nuclear syntheses.
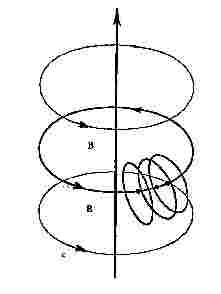
Figure 7.8. A schematic view of the geometry of a DC electric arc represented by the vertical line with the associated magnetic field represented by horizontal circles, and the created magnecules represented by circles perpendicular to the magnetic lines. This geometry has the following primary implications: 1) Since the magnetic field M is proportional to
I / r, one can see that at atomic distances from electric arcs with one thousand Amperes of current, the magnetic field is of the order of 1011 Gauss, thus being sufficient to polarize atomic orbitals into toroids, and then coupling them into magnecules.
In figure 7.9, we provide the conceptual lines of hadronic refineries of current industrial production consisting of a metal vessel capable of withstanding pressures up to 300 psi; the vessel is filled up with the selected liquid feedstock, and houses a DC electric arc among one or more pairs of electrodes that are submerged within the selected fluid and feedstock powered by an external AC-DC converter with at least 50 Kw. Electrodes are selected depending on the need at hand. For instance, in the event carbon is needed to stabilize the fuel produced or for nuclear syntheses, the electrodes can be composed of commercially available graphite.
The equipment includes means for the continuous recirculation of the liquid feedstock through the arc so that magnecular structures are continuously removed from the arc following their creation. In the case of a liquid feedstock, the submerged arc produces a clean burning, cost competitive magnecular fuel that bubbles to the surface where it is collected for use (see web site of the U. S. public company Magnegas Corporation, www.magnegas.com and, or additional technical details, one may inspect Santilli's various U. S. Patents in the process known as PlasmaArcFlow.).
The heat produced by the reactor is acquired by the liquid feedstock and it is used via its recirculation through an external heat exchanges that can power a turbine for the production of electricity (following additional input to the steam to reach supercritical temperatures) or other uses. The equipment is completed by means for the automatic control of all operations, including the control of the DC power, the pressure, temperature, flow, and other means.
The efficiency of hadronic refineries is quite big and not entirely understood until now. In essence, the arc turns the liquid feedstock into a gaseous state; decomposes the gas molecule into their atomic constituents; ionizes the resulting atoms; and forms a plasma at about 10,000 degrees F composed of ionized H, O, C and other atoms, ionized dimers OH and CH, and ionized ordinary molecules. The known affinity between C and O creates C-O in single, double and triple bonds, the combustion in part of C-O into CO2, the synthesis of H-H and other esoenergetic reactions.
Because of the above features, Santilli's hadronic refineries provide a new form of carbon combustion that is cleaner and more efficient than conventional carbon combustion, e.g., in the boiler of an electric power plant. In fact, the produced gaseous fuel is cleaned via its passage through the liquid feedstock. the trapped impurities being recycled by the arc, thus providing a form of carbon combustion cleaner than existing combustion. Santilli's carbon combustion is also more esoenergetic than conventional forms for the evident reason that the latter solely allow conventional chemical reactions for CO, CO2 and other lesser relevant reactions, while the former admits additional very esoenergetic reactions such as the synthesis of H2 as well as of magnecules.
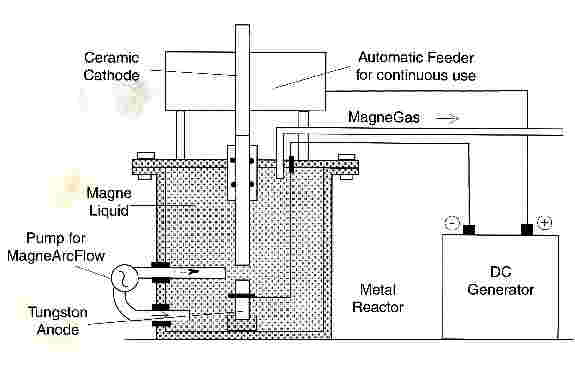
Figure 7.9. The main lines of Santilli hadronic refineries converting liquid waste into a clean burning, cost competitive gaseous fuel with magnecular structure, showing: the pressure metal vessel; the submerged electrodes; the recirculation of the feedstock through the arc; the external AC-DC converter; the external automatic controls of the arc; and the collection of the produced magnecular fuel.
The existence of clearly anomalous species is easily established Santilli hadronic refineries because in using pure graphite electrodes within distilled water, the maximal species predicted by quantum chemistry is CO2 with 44 amu, while clusters in macroscopic percentages are detected all the way to 500 amu, with detection and all the way to 1,000 amu in lesser percentages, which clusters can only be Santilli magnecules. Additional large deviations from quantum chemistry are visible in the exhaust and other aspects.
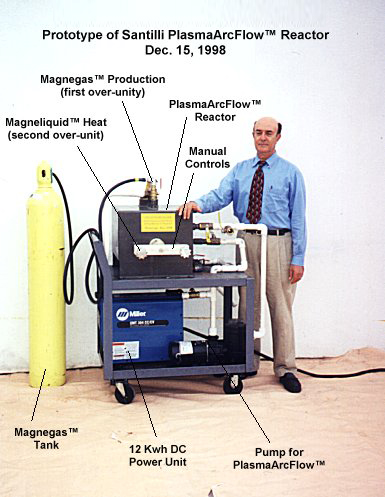
Figure 7.10. Picture of Prof. Santilli with with the prototype of hadronic refineries he built in Florida in late 1998.
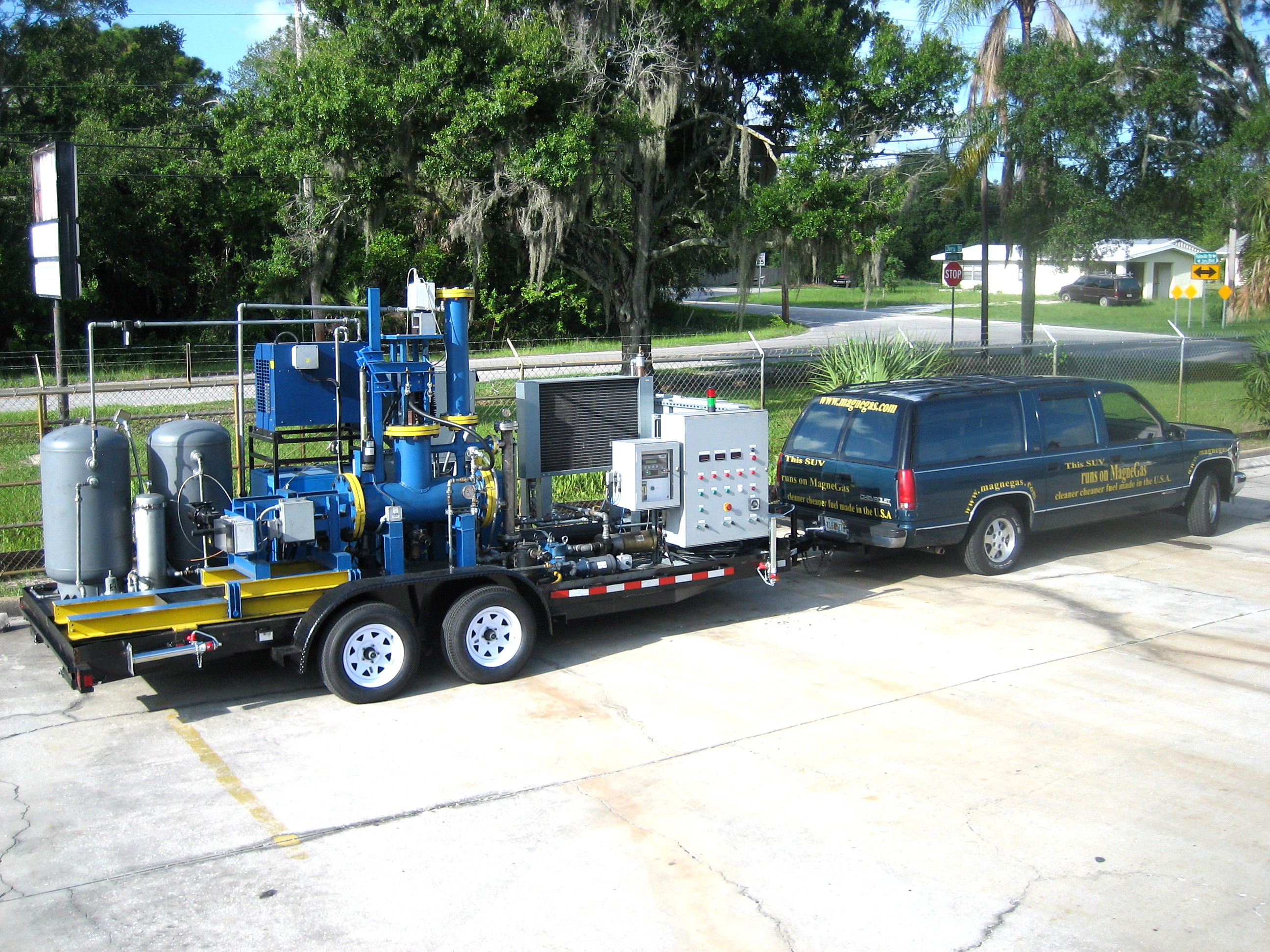
Figure 7.11. Picture of a mobile 50 Kw hadronic refinery pulled by an SUV built by Prof. Santilli in summer 2005.
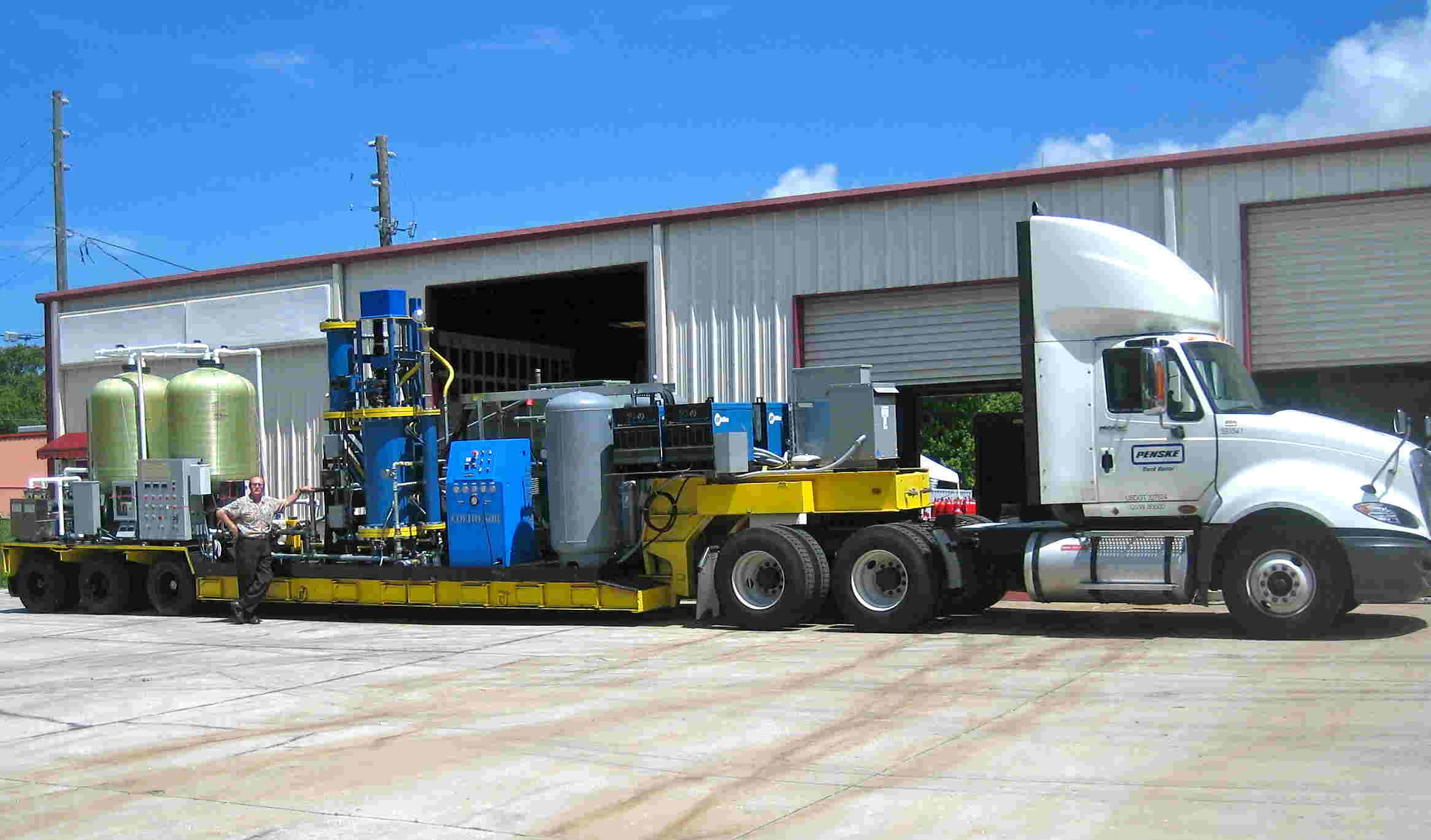
Figure 7.12. Picture of Prof. Santilli with an industrial hadronic refinery delivered to China in Spring 2010.
To conduct measurements, Santilli [loc. cit.] has introduced first the Scientific Efficiency (SE) defined as the ratio between the energy output (given by the heat energy contained in magnegas Emg plus the heat acquired by the liquid feedstock Efs) and the energy input (given by the electric energy Eel used by the refineries plus the energy released by carbon combustion and the other above indicated reactions Ecc),
(7.22) SE = (Emg + Efs)/ (Eel + Ecc) < 1,
which efficiency is evidently smaller than one because of the conservation of the energy, dispersions and other reasons.
However, oil waste is very rich in carbon and its recycling not only has no cost, but actually brings an income. For this reason, Santilli introduced the Commercial Efficiency (CE) given by the preceding definition with the sole electric energy in the denominator since it is the sole carrying a cost,
(7.23) SE = (Emg + Efs) / Eel > 1.
The latter efficiency can be greater than one, as established by certified and independent measurements, because the energy produced by the combustion of the carbon in the plasma of the arc is much bigger than the electric energy used by the arc. Note that this value bigger than one occurs also in using distilled water as a feedstock since the carbon is supplied by the graphite electrodes.
As an illustration with data verifiable at the laboratory of the Institute for Basic Research in Florida, a hadronic refinery operating with 100 Kw, 100 psi pressure, and 200 degrees F for the recycling of an oil-base liquid feedstock (such as automotive oil waste, biodiesel byproducts, frying oil waste, etc.) produces magnegas at the rate of about about 1,500 scf/h = 42,000 ~L/h corresponding to about $1,350,000 BTU/h, plus heat acquired by the liquid feedstock of about 600,000~BTU/h, while using 100~ Kwh that correspond to about 340,000 BTU/h. In this case the scientific efficiency is, evidently, smaller than one, but the commercial efficiency is bigger than one and given by
(7.24) SE = (Emg + Efs) / Eel =
(1,350,000 + 600,000) / 340,000 = 5.73.
which value establishes the industrial relevance of hadronic refineries due to their large efficiency.
The reader should be aware that commercial efficiencies (as above defined) of the order of 10 have already been measured in prototypes because the total net energy output of hadronic refineries increases nonlinearly with the increase of the operating power, pressure and temperature.
7.3J. The physics of intermediate controlled nuclear fusions
The physics of ICNF was first presented by Santilli in the 1998 monograph, studied from a chemical viewpoint in the 2001 monograph, and then finalized in the 2008 memoir. In this section we review these studies based the following main assumptions:
ASSUMPTION I: The synthesis of neutrons from protons and electrons is the first and most fundamental synthesis in nature. Nuclear fusions can only follow that of the neutron. Hence, no nuclear fusion is expected to occur at energies intermediates between the "cold" and the "hot" fusions unless reactors are capable of achieving the neutron synthesis. Consequently, ICNF should take into consideration possible contributions from the synthesis of neutrons from protons and electrons.
ASSUMPTION II: Quantum mechanics is fundamentally inapplicable to the neutron synthesis as well as that of all nuclear syntheses at large due to its reversible structure compared to the irreversibility of the syntheses considered and for many other reasons (Chapters 1, 3, 6). Consequently, any appraisal of nuclear fusions basically dependent on quantum descriptions cannot be final.
ASSUMPTION III: Operator mechanics applicable to nuclear fusions should first achieve a time invariant numerical representation of all characteristics of the fundamental synthesis of neutrons from protons and electrons, as a condition to be applicable to subsequent nuclear syntheses. Hadronic mechanics is the only mechanics verifying these pre-requisites to our knowledge at this writing. Other theories are dismissed by Santilli unless: 1) they achieve said "numerical" representation of "all" characteristics of the neutron; 2) said representation is invariant over time (predict the same numbers under the same conditions at different times); and 3) is proved to be inequivalent to hadronic mechanics.
The guidelines for the conception of hadronic reactors has been based on nature, rather than on pre-existing research. As established by chemical analyses of air bubbles in amber, about one hundred millions years ago Earth's atmosphere had about 40 % nitrogen, while its current percentage is about double that value. Other chemical analyses show that the increase of nitrogen in our atmosphere has been gradual.
In Santilli's view, these data suggest the apparent existence in our atmosphere of a process causing the natural synthesis of nitrogen from lighter elements. Since nature is notoriously friendly toward the environment, such a process is expected to synthesize nitrogen without the release of of harmful massive radiation such as neutrons, protons and alpha radiations, from, which ICNF Law VI was derived.
Among all possible origins of a nitrogen synthesis in our atmosphere, the most probable one is given by lighting, because a serious scientific (that is, quantitative) explanation of thunder cannot be achieved with conventional physical and chemical reactions, thus requiring nuclear syntheses. In fact, a numerical explanation of thunder requires energy equivalent to hundreds
of tons of explosives that simply cannot be explained via conventional processes due to the very
small cylindrical volume of air affected by lightning plus its extremely short duration of
the order of nanoseconds.
The nitrogen syntheses by lighting provides indeed a numerical explanation
of thunder as well as the slow rate of nitrogen increase in our atmosphere. Among all possible syntheses, the most probable one results to be the synthesis of nitrogen from carbon and deuterium. However, the deuterium presence in our atmosphere is excessively small to permit a numerical explanation of thunder. It is at this point where the synthesis of neutrons by lighting from protons and electrons enters rather forcefully into the arena of nuclear syntheses.
In fact, the neutron synthesis is expected to be a necessary pre-requisite for the synthesis of deuterium in atmosphere that, in turn, allow nitrogen syntheses with values sufficient to reach a numerical explanation of thunder. At any rate, Santilli succeeded in synthesizing neutrons from protons and electrons precisely via the use of an electric discharge in a hydrogen gas (Chapter 6). Needless to say, numerous additional fusions are also possible under lighting and some of them will be indicated below.
Hadronic reactors have been conceived to reproduce lighting processes within a gas as close as technically possible at the moment. In the authors view, the biggest unknown at this writing is not given by the identification of possible nuclear syntheses triggered by lighting, but by our insufficient knowledge of lighting itself due to departures from quantum mechanics while studies via the covering hadronic mechanics are not yet completed at this writing.
The successful achievement of ICNF with industrial relevance crucially depends on the proper selection of the hadronic fuel In this section, we study a few examples of hadronic fuels selected under the conditions that:
1) The original and final nuclides are light, natural, and stable isotope;
2) The nuclear syntheses cause no emission of harmful radiations, such as n, p, α, etc.; and
3) The energy produced Δ E is much bigger than the total energy used by the equipment for its production.
Note the difference between the conventional nuclear fission, where the emphasis is in the use of large, often unstable isotopes, and the proposed novel hadronic energy, where the emphasis is in the opposite selection of isotopes as light and stable as possible. As indicated earlier, the latter emphasis is necessary to achieve a basically novel nuclear energy without harmful radiations and without harmful waste. Santilli has shown that these main objectives are indeed realistic, not via the use of quantum theories, but via the use of the covering hadronic mechanics and its laws for.
By using standard nuclear terminologies and symbols with: A, Z, Jp, u denoting the atomic number, the nuclear charge, the nuclear angular momentum, the parity, and the nuclear energy in amu units, respectively, the ICNF proposed buy Santilli are of the generic type
(7.25a) N1(A1, Z1,J1p1, u1) + N2(A2, Z2, J2p2, u2) + TR →
→ N3(A3, Z3, J3p3, u3) + Heat,
(7.25b) A1 + A2 = A3, Z1 + Z2 = Z3, J1 + J2 = J3, p1 + p2 = p3,
(7.25c) Δ E = E3 - (E1 + E2) > 0,
where E is measured in units "u", TR denotes the "trigger" and the heat is essentially produced by the release of excited states of the synthesized nucleus N3 under energies insufficient to produce massive radiations.
It should be stressed that a number of alternatives to the above synthesis are possible, particularly those based on intermediates processes such as Electron Capture (EC), or emission of electrons that is not considered harmful since electrons can be stopped with a thin metal shield. Needless to say, in the latter cases, the conservation of the charge is different than that in Eq. (7.25).
The following unit conversions may be helpful:
(7.26 ) 1 u = 931.494 MeV; 1 MeV = 1.602 x 10-13 J =
= 4.45 x 10-17 Wh = 1.511 x 10-16 BTU;
(7.26b) 1 Wh = 3.397 BTU; 1 C = 6.241 x 1018 e; 1 A = 1 C / 1s,
where "e" is the elementary charge of the electron. The energy used by a 50 Kwh hadronic reactor is given by
(7.27) 50 Kwh ≈ 1.69 x 105 BTU.
Hence, to be industrially relevant, hadronic reactors must produce energy at an hourly rate much bigger that that used for their operations.
Santilli then first studies the synthesis of nitrogen as excepted in lighting. The simplest reaction with related energy output is given by:
(7.28a) C(12, 6, 0+, 12.0000) + H(2, 1, 1+, 2.0141) →
→ N(14, 7, 1+, 14.0030) + Heat,
(7.28b) Δ E = (EC + EH) - EO = 0.0111 u = 10.339~ MeV ≈ 1.5 x 10-15 BTU,
where very light elements, such as hydrogen and helium, are expected to be completely ionized at the intermediate energies needed for the ICNF, namely, H(1, 1, 1/2+, 1.0078) ~ = p, H(2, 1, 1+, 2.0141) = α, etc., an assumption tacitly implemented hereon. Note that the preceding reaction verifies conventional nuclear conservation laws and can be engineered to verify all additional CNF laws.
The above synthesis is indeed of expected industrial relevance because the hourly rate of 1030 ICNF, a rather reasonable expectation due to the volume of available gas, would overcome the used energy and yield the hourly production of about 1010 BTU, namely, a rather significant new clean energy patterned along natural lighting.
Fusion (7.28) is more deeply interpreted as originating from the following two-steps process
(7.29) C(12, 6, 0+, 12.0000) + 2 x H(1, 1, 1/2+, 1.0078) + TR + EC + a →
→ C(12, 6, 0+, 12.0000) + H(2, 1, 1+, 2.0141) + TR →
→ N(14, 7, 1+, 14.0030) + Heat,
namely:
1) The electric arc first polarizes the carbon and hydrogen atoms by forming the magnecule C x H x H, including the necessary triplet axial coupling of spins.
2) Under a suitable trigger, the magnecule C x H x H can only yield a nucleus with A = 14, Z = 8, Jp = 1+ that is known not to exist (since O(14, 8) has spin J = 0). The sole electron capture (EC) would also yield a nucleus known not to exist.
3) The explanation adopted by Santilli is that nature synthesize the neutron from protons, electrons and etherinos according to the sequence:
(7.30) C x H x H → C(12, 6, 0) + 2 x p + e + a →
→ C(12, 6, 0) + H(2, 1, 1) → N(14, 8, 1).
where we assume the correct spin couplings requested for ICNF. Another ICNF also suggested by lighting is given by
(7.31) O(16, 8, 0+ 15.9949) + H(2, 1, 1+, 2.0141) + TR →
→ F(18, 9, 1+, 18.0009),
(7.31b) Δ E = 0.0081 u = 7.545 MeV,
and secondary process due to the instability of F(18, 9, 1+, 18.0009)
(7.32) F(18, 9, 1+, 18.0009) + EC → O(18, 8, 0+, 17.9991) + 1.656 MeV,
resulting in the following total energy output per synthesis
(7.33) Δ E = 9.201 MeV ≈ 1.30 x 10-15 BTU,
in which case, again, 1030 syntheses per hour would yield a rather substantial new clean energy.
An additional selection of hadronic fuel is a 50-50 mixture of deuteron and helium gases according to the following ICNF
(7.34a) H(2, 1, 1+, 2.0141) + He(4, 2, 0+, 4.0026) + TR →
→ Li(6, 3, 1+, 6.0151) + heat
(7.34b) Δ E = 0.0016 u ≈ 2.5 x 10-16BTU,
that verifies all CNF laws. Hence, one can see that a hadronic reactor with the above hadronic fuels becomes industrially relevant under the achievement of about 1030 CNF per hour, that would yield the hourly production rate of about 109 BTU.
Note the need, again, of deuterium for ICNF (9.15) and (9.18) and the need that deuterium be synthesized following the prior synthesis by lighting of the neutron. In turn, as the reader with serious interest in new knowledge will see, these aspects have a crucial relevance for the achievement of ICNF with industrially valuable energy output.
Another ICNF based on lithium is given by
(7.35a) Li(7, 3, 3/2-, 7.0160) + H(1, 1, 1/2+, 1.0078)
+ TR →
→ 2 x He(4, 2, 0+, 4.0026),
(7.35b) Δ E = 2.887 x 10-12 J,
where one should be aware of the opposing nuclear polarizations needed to verify the law on the conservation of the angular momentum, a feature of crucial relevance for the engineering realization to have any hope of achieving industrial relevance.
The energy output of reactions (9.20) is significant. by using one mole of lithium that has 2023 nuclei; by assuming an efficiency of 1016 per minute; and by using energy units in Joules, we have the energy output
(7.36) Δ E 2.8 x 104 J/min = 1.7 106 J/h.
that is indeed industrially relevant.
At this point it is important to identify the rather dramatic differences, with negative environmental implications, between a number of studies via the "cold fusion" and the proposed ICNF. A typical reaction believed to be necessary for the "cold fusion" of two deuterium nuclei, is that into the tritium plus the emission of a neutron,
(7.37) H(2,1, 1+, 2.0141) + H(2,1, 1+, 2.0141) + TR →
→ He(3, 2, 1/2+, 3.0160) + n.
from which one can see the judgment on the existence of "cold fusion" of two deuterium nuclei on whether neutrons are emitted or not.
It is essential for the protection of the environment to show that the above criterion to appraise "cold fusions" is a pure theology if used as a basis to deny their existence. In fact, the belief is based on the need to conserve the total angular momentum that normally would yield the value J ~= ~2 for the l.h.s of reaction (7.37). However, hadronic mechanics does indeed admit the synthesis of He-4 from two deuterium nuclei without any emission of massive radiation, yet via the appropriate use of deuterium nuclei with opposing polarizations according to the law
(7.38) H(2,1, 1+↑, 2.0141) + H(2,1, 1+↓, 2.0141) + TR →
→ He(4, 2, 0+, 4.0026) + heat,
where one should keep in mind, again, the necessary spin couplings for the reaction to occur.
The engineering realization of the latter reaction is studied in the next section.
At this stage we limit ourselves to indicate that the denial of nuclear syntheses based on the lack of neutron emissions is purely political and without serious scientific foundation, particularly when proffered by experts, because the latter are expected to know possibility (7.38) to qualify as such. It is at this point where problems of scientific ethics also emerge forcefully as a prerequisite for the solution of our serious environmental problems.
The difference between pre-existing studies on "cold fusion" and the proposed ICNF is also illustrated by the well known setting in which the reactor is filled up with
hydrogen, and the electrodes are made up of palladium 106. In these cases we should have fusions inside the palladium cathode of the type
(7.39) Pd(106, 46, 0+,105.9034) + H(1, 1, 1/2+, 1.0078) + TR →
→ Ag(107, 47, 1/2+ 106.90509).
The above reactions do verify conventional nuclear conservation laws. However, the engineering implementation of the new CNF laws inside the palladium electrodes is virtually impossible, thus explaining the reason for the lack of consideration in the industrial research herein reported. The above occurrences also illustrates the viewpoint expressed from Section 1 to the effect that the author believes that nuclear fusions do indeed occur in setting of the type (7.39). However, they can at best be at random, thus precluding a serious hope to achieve the controlled energy output necessary for industrial relevance.
7.3K. Engineering conception of hadronic reactors
By using all the preceding advances, Santilli proposed specific and concrete engineering realizations of hadronic reactors first presented by in the 1999 monograph, then revised in the 2001 monograph and finalized in the 2008 memoir. We begin with a generic embodiment suitable for a variety of hadronic fuels, and then pass to more specific reactors with specific hadronic fuels.
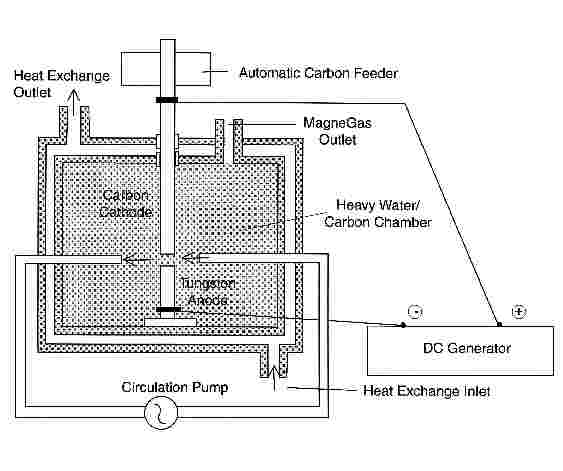
Figure 7.13. A schematic view of the Hadronic Reactor, essentially based on an engineering upgrade of the Hadronic Refineries of the preceding figures, showing the shift of emphasis, from the production and use of a magnecular fuel in the latter, to the production and use of heat in the former.
Figure 7.13 presents a conceptual outline of a simple hadronic reactor in which the primary differences with the hadronic refineries of the preceding section are: refineries use a liquid feedstock, while reactors generally used a gaseous feedstock; refineries operate at generally low pressures of the order of 30 psi, while reactors need to operate at high pressures of the order of 3,000 psi or more to achieve industrial relevance due to the decrease of density of about 1,000 in the transition of the feedstock from a liquid to a gas; and reactors need heat exchangers much more efficient than refineries due to the decrease in heat propagation from liquids to gases.
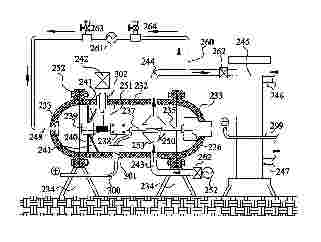
Figure 7.14. A more detailed view of a preferred embodiment for the industrial realization of hadronic reactors proposed for the industrial realization of ICNF.
In more engineering details with respect to Figure 7.14, from one of Santilli's we recall that the proposed hadronic reactor
comprises: a metal vessel 232 with hemispherical heads 233, fasteners 252 and bases
234 capable of withstanding a pressure of at least 10,000 psi (666 bars); a stationary, negatively charged, anode 235 hat protrudes outside the hemispherical head 233 for connection via cable 299 to the negative polarity of a steady or pulsing AC-DC converter with at least 50 Kw power (not shown in the figure), said protrusion occurring through insulating pressure resistant bushing 236 in phenolic
G10 or equivalent material; an internally movable, positively charged cathode 237 connected via
cable 300 and insulating bushing 301 to the positive polarity of said outside power
source; said cathode 237 being connected via insulating phenolic G10 block 238 to a metal rod
equipped with rake 239 that is internally fastened to vessel 232 via brackets 240; said rake 239
being operated by a pignon 240 that is controlled by an outside servomotor 242 through
insulating pressure resistant thrust bearing 302; vessel 232 being filled up with a gaseous or liquid
feedstock 251 that is recirculated through the electric arc 250 via pump 252 through
pipe 253; the feedstock is then forced via pipe 244 for passage through heat
exchanger 245 for continuous recirculation through the arc 250 via pump 252 and pipe
253; the heat acquired by heat exchanger 245 being utilizes via an external fluid via
inlet 246 and outlet 247; the proposed hadronic reactor being completed by pipe 248 for
burst of pressure of the gaseous feedstock inside vessel 232 to realize the hadronic
trigger, said burst of pressure being realized by outlet 260 and impact blower 261,
check valves 262 protecting the primary pump 252 and the heat exchanger 245.
The operation of the proposed hadronic reactor is the following. Firstly, a vacuum inside
vessel 232 is secured via valve 263. Subsequently, valve 263 is closed and the vessel is filled up
with feedstock 251 via valve 264 up to the preset pressure of at least 5,000
psi (333 bars) for gases and up to $2/3$ of the interior volume for liquids. Upon completion of the filling operation, the automatic controls
activate the AC-DC converter and the primary pump 252 with the continuous recirculation of the
feedstock through the arc. When the electrodes are at such a distance not allowing an arc for the pre-selected feedstock and for the pre-selected pressure (open arc), the automatic controls activate servomotor 242 acting
on pignon 240 that activates rake 239 solidly connected to cathode 237 via
insulating bushing 238, to move said cathode 237 toward the
stationary anode 235 until such a distance at which an electric arc of high current (e.g., 1,000 A)
within said feedstock is initiated. This first phase serves to create magnecules. The
automatic controls then increase the gap between the electrodes to such a value for which the
variation of the voltage is within preset values (one of the twenty adjustable parameters of the
automatic controls of the Magnegas Technology [9d]), so as to maximize the voltage, or the gap between the electrodes, or the travel of the arc within
the feedstock. Following a preset duration of
such high current arc, the automatic control activate the high voltage impulse current as a partial
realization of the trigger. According to a pre-set frequency, the automatic control also activate the
impulse pump 261 to create burst of very high pressure inside vessel 232. This provides an engineering realization of the trigger via a
combination of the following three means: 1) Impulse high voltage arcs; 2) Impulse high
pressures; and 3) the enhancement of both preceding contributions by the arc geometry (Figure 5).
It would be naive to assume that the above description is exhaustive, since numerous other features
are needed to render the above hadronic reactor industrially viable. The author regrets not to have received the necessary authorization by the investors for their disclosure at this writing.
1. Hadronic nitrogen reactor. The engineering realization of ICNF for reaction (7.28) is conceptually simple. It is given by a reactor with general lines depicted in Figure 7.13 and engineering realization studied in the next section. The metal vessel is filled up with deuterium gas at 3,000 psi pressure that is recirculated through graphite electrodes. The trigger is realized by pulse DC with with 100,000 V and 5 mA and other means we are not authorized to disclose at this time. The heat is dissipated by the external heat exchanger.
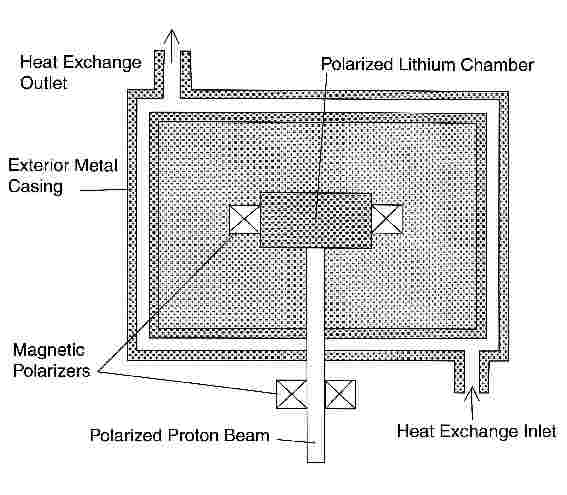
Figure 7.15. A schematic view of one of the simplest hadronic reactor, that for the synthesis of the lithium as per Eqs (7.34).
2. Hadronic oxygen reactor: The engineering realization of ICNF (7.31) is, conceptually, one of the simplest because the reaction does not require spin polarizations for the conservation of the angular momentum. Hence, it is sufficient to use the embodiment conceptually outlined in Figure 13. The metal vessel is filled up with a 50-50 mixture of oxygen 16 and helium at 3,000 psi, which mixture is recirculated through a 50 Kw electric arc between carbon electrodes, thus creating magnecules of the type OxHe. The trigger can be given by DC pulses with 100,000 V and 5 mA, or by impulse pressures and other mechanisms. The heat produced is absorbed by the external all encompassing vessel and utilized via heat exchangers.
3. First hadronic lithium reactor: The engineering realization of the first lithium reaction, Eq. (7.34), is essentially the same as that for the oxygen reactor, the only difference being that the vessel is filled up with a 50-50 mixture of hydrogen and helium gases also at 3,000 psi. The mixture is also recirculated through a 50 Kw electric arc that creates magnecules HxHe. The trigger can also be given by a high voltage pulse DC current or impulse pressure or other mechanism.
4. Second hadronic lithium reactor: The engineering realization of the second lithium reaction, Eq. (7.35), is more complex than the preceding one because of the need of lithium nuclei and a beam of protons with opposite polarization as a necessary condition to avoid a random reactions as occurring in "cold fusions". Current technology allows a variety of engineering realization of the needed polarization represented in Figure 7.16 where a proton beam with down polarization enters a chamber of lithium with up polarization, both polarizations being realized via magnetic fields. The efficiency of the hadronic reactor is then dependent on the geometry of both the proton beam and the lithium chamber plus an adequate trigger the author has not been authorized to disclose here at this time.
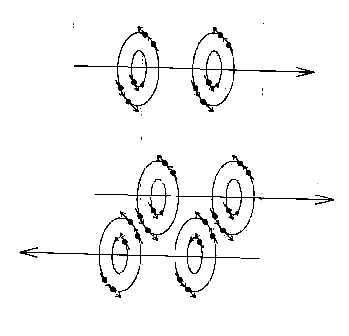
Figure 7.16. A schematic view of the singlet (antiparallel) spin coupling necessary to synthesize the helium from deuterium according to nuclear synthesis (7.38) which is of evident very difficult engineering realization for controlled systematic fusions.
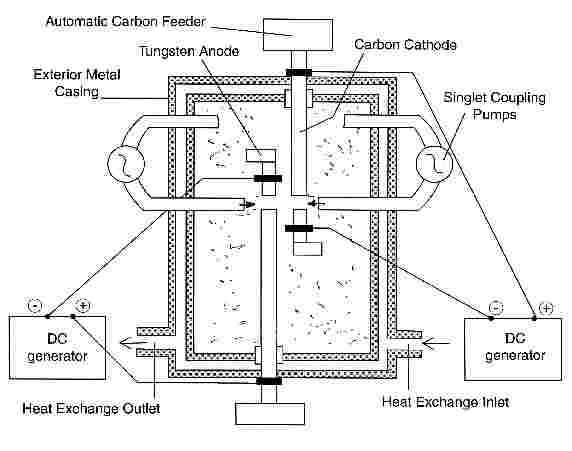
Figure 7.17. A schematic view of one of the most difficult hadronic reactors, that for the synthesis of the helium from two deuterium atoms with opposing polarization.
5. Hadronic helium reactor: The engineering realization of reaction (7.38) is one of the most difficult because it requires the application of a trigger to two beams of deuterium gas with opposite spin polarizations. The main idea expressed in the embodiment of Figure 7.17 is that of a metal vessel as in the preceding reactors that houses two parallel but separate electric arcs with opposing polarities so as to produce opposite polarizations of said deuterium gas. The flow of the gas through said arcs from opposite directions then creates the superposition of said beams in the area located between said arcs with spin couplings depicted in Figure 7.17. In this case the trigger may be given by impulse pressure or other means.
7.3L. Experimental verification of nitrogen synthesis without harmful radiations or waste
7.3La. Experimental set up
In this section, we review Santilli's 2010 paper presenting one of the most important experimental verifications of the entire mathematical, physical and chemical research studied in this volume, verification conducted in early 2010 at the laboratory of the The Institute for Basic Research (IBR) in Tarpon Springs, Florida, and consisting of the apparently first achievement, per our knowledge, of the nuclear synthesis of nitrogen from carbon and deuterium without any release of harmful radiation or radioactive waste, according to reactions (7.28).
With reference to Figure 7.18, Santilli constructed in early 2009 a hadronic reactor consisting of a 12" diameter and 24" long Schedule 80 carbon steel pipe with related flanges, tested at 300 psi so as to safely operate at 150 psi pressure, equipped with internal electrodes composed of commercial grade graphite, the anode being stationary and the position of the cathode being controllable from the outside via a suitable insulated knob allowing the initiation and disconnection of the arc, said reactor being completed by inlet and outlet gaseous ports, pressure and other gauges.
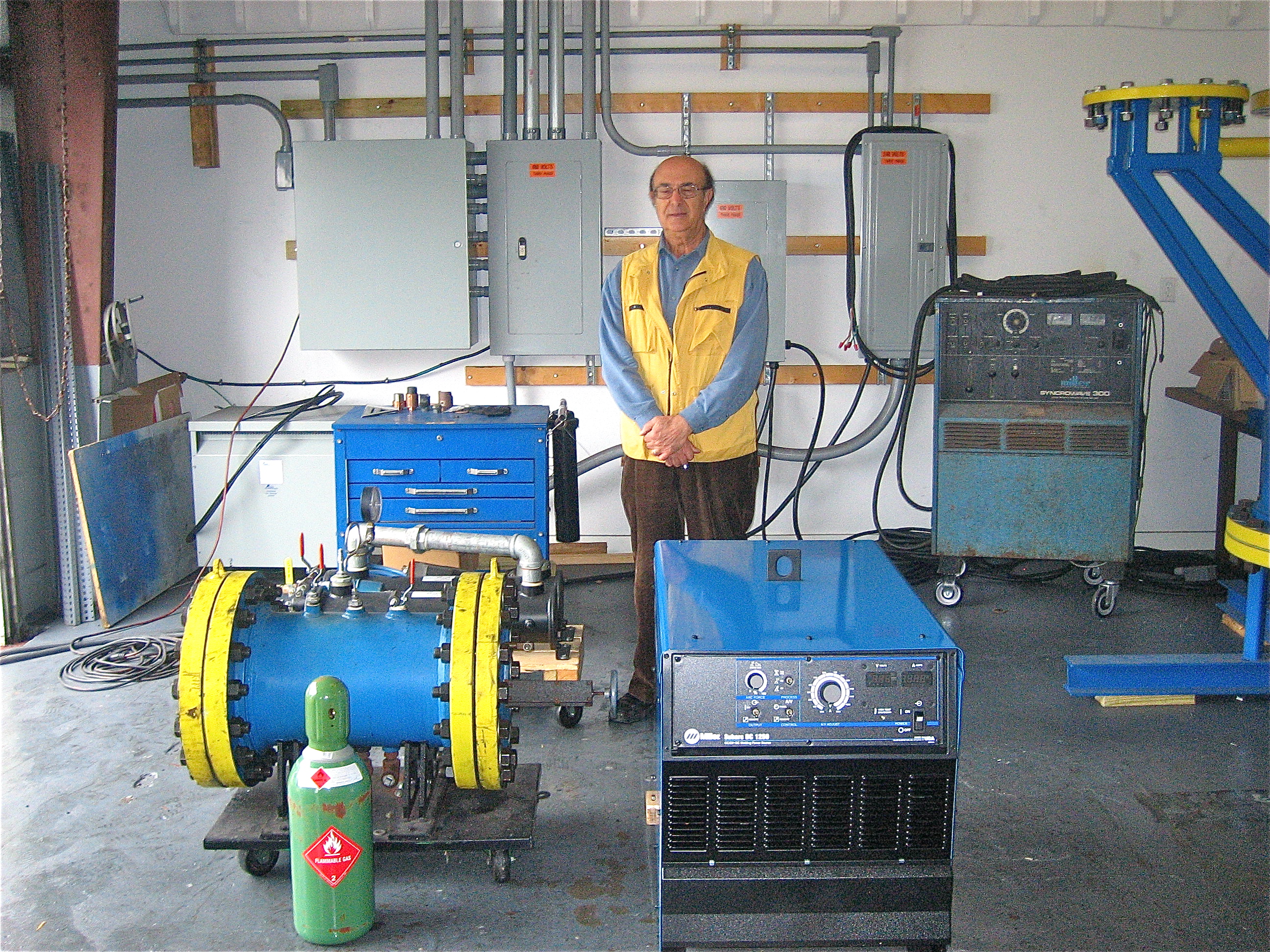
Figure 7.18. A view of Prof. Santilli with the equipment used for the synthesis of nitrogen from carbon and deuterium showing fro the r.h.s.:. the Miller Dimension 1000 AC-DC converter; the pressure bottle of 99.99 pure deuterium; and the 12" x 24" schedule 80 hadronic reactor.
Radiation counts during the test were done via the following detectors placed next to the hadronic reactor:
1) A photon-neutron detector model PM1703GN manufactured by Polimaster, Inc., with sonic and vibration alarms as well as memory for printouts, with the photon channel activated by CsI and the neutron channel activated by LiI;
2) A photon-neutron detector SAM 935 sold by Berkeley Nucleonics, Inc., with the photon channel activated by NaI and the neutron channel activated by He-3 also equipped with sonic alarm and memory for printouts of all counts;
3) A BF3 activated neutron detector model 12-4 manufactured by Ludlum Measurements, Inc., without counts memory for printouts but with both visual and sonic means;
4) An alpha, beta, gamma and X-ray detector model 907-palmRAD sold by Berkeley Nucleonics, Inc.; and
5) Various material suitable for nuclear transmutations.
7.3Lb. Conduction of the tests.
On January 7 2010, a vacuum was first pulled out of said reactor by IBR technicians Gene West and Michael Rodriguez who subsequently filled up the reactor up to 100 psi with deuterium gas 99.99 % pure as certified and supplied by Advanced Special Gases of Reno, Nevada. The original deuterium tank was then disconnected. A two-valves laboratory bottle market HT1 was then filled up for chemical analysis with the gas in the interior of the reactor, thus including the original deuterium plus internal impurities. Commercially available digital sensors were used for the recording of temperatures, pressures, times and other data.
No PlasmaArcFlow of the deuterium gas through the arc was activated because the first reactor here referred to did not have a cooling system and, in its absence, operations had to be stopped in one or two minutes due to excessive production of heat. Also, the experiment was intended solely to establish the existence of nuclear synthesis (7.28) with the absence of harmful radiation or waste, since the achievement of a large scale production of energy requires basically different approaches and vast development funds in due time.
Still, with reference to Figure 7.18, the electrode terminals of the reactor were connected to a commercially available Miller Electric Dimension 1000 AC-DC converter set to operate at 40 Kwh. Gene West and Michael Rodriguez activated the DC electric arc in the interior of the reactor for two minutes, after which time the arc had to be disconnected because the reactor, originally at about 20o C and 100 psi, had reached well over 150o C and 200 psi, with the external paint showing signs of scorching.
A second two-valves laboratory bottle was marked HT2 and filled up with the gas in the interior of the reactor following the activation of the arc due flushing. Under the trail of custody by the IBR technician Jim Alban, the two laboratory bottles so obtained were shipped to ORS Oneida Research Services of Whiteboard, New York, for analyses.
7.3Lc. Experimental results.
The first and perhaps most important feature reported in the 2010 paper, under the eye witnessing of Gene West, Michael Rodriguez and Jim Alban, is the absence of any massive radiations in the outside of of the hadronic reactor, with particular reference to the absence of any detection of neutrons that, in case produced, are predicted to be detectable in the outside.
Repeated tests of the interior of the reactor following the tests showed complete absence of any radioactive waste. Internally produced charged particles are easily absorbed by the thick Schedule 80 metal walls of the reactor and cannot be detected in the outside. In any case, no production of neutrons, protons or alpha particles is possible due to insufficient energies for the fission of light stable nuclei.
The analyses on samples HT1 and HT2 were conducted by ORS Oneida Research Services, via an Internal Vapor Analyzer, model 110-s which is the latest version of the system. The analyses were performed per ORS SOP MEL-1070,Gas Analysis of Sealing Chamber Atmosphere. The main results are shown in Figure 7.19.
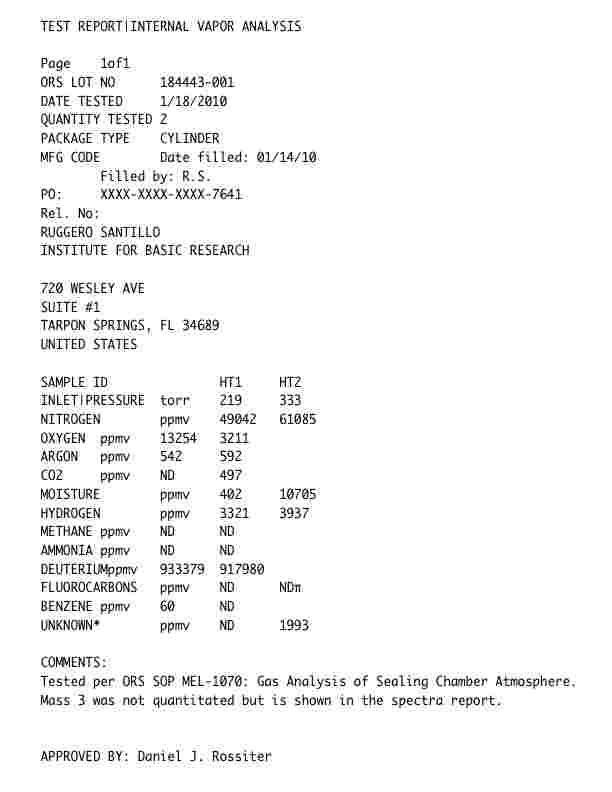
Figure 7.19. A view of the the results of the analysis of samples HT1 and HT2 by ORS Oneida Research Services showing a clean increase of nitrogen counts combined with a clean decrease of deuterium counts.
The most significant measurements (see Figure 7.19) are the decrease of the deuterium gas in two minutes of operation from 93.3 % to 91.8 % and the increase of nitrogen has from 4.90 % to 6.11 %, thus providing experimental confirmation of the nitrogen synthesis (7.28) as predicted in the 2008 memoir.
Heat measurements were done as follows. The reactor essentially consisted of a schedule 80 pipe 1 ft diameter and 2 ft long, with two hollow flanges welded at its ends. According to tabulated data (verified with approximate but actual measurements), the weight of this assembly is of 325~lbs plus the weight of the steel in the four (two external and two internal) weldings. To be conservative, Santilli assume that the weight of this assembly, hereon referred to as the "cylindrical component," is of 300 lbs.
Additionally, the reactor comprises two plain flanges each having a tabulated (and approximately but actually verified) weight of 189 lbs. However, these flanges are thermally isolated from the cylindrical component to a great extent due to the gaskets between the plain flanges and the cylindrical component. In fact, systematic heat measurements showed that the cylindrical component would acquire heat much faster and in much greater amount than the terminal flanges.
Consequently, the heat measurements were solely referred to the cylindrical component. The measurements on the terminal flanges were deferred to future independent verifications since, as indicated above, the primary objective of the 2010 paper is to ascertain the existence of ICNF, and not their industrial output.
As also indicated earlier, the tests on deuterium gas at 100 psi with a 40 kW arc between carbon electrodes operated for two minutes showed a systematic increase in temperature, from the ambient temperature in the range of 20o C to generally over 150o C with a conservative average increase of about 127o C, The use of the known expressions (449J/kg.C x 136.077kg x 127C)/1055.06J/BTU yields the heat acquired by the cylindrical component (cc)
(7.40) ΔEcc = 7404 BTU,
By recalling the known value 1 kwh = 3400 BTU, the use of 40 Kwh for two minutes yields
(7.41) ΔEarc = 4533 BTU.
Consequently, the nuclear syntheses in the interior of the reactor yielded in two minutes the excess heat of
(7,42) ΔEout = 2,871~ BTU,
by keeping in mind that Santilli did not considered the heat acquitted by the isolated side flanges. Consequently,l the actual value of the excess heat is bigger than the above, thus confirming the existence of a significant internal source of energy beyond that of the AC-DC converter.
Recall that a vacuum was pulled out of the reactor, that was then filled with 99.99 % pure deuterium gas which, prior to the test, measured 93.3 %, the residual gases being noncombustible except for traces of oxygen. Consequently, the excess energy release cannot possibly be attributed to internal combustion since the deuterium gas is not combustible when alone. Hence, the sole credible interpretation is that the excess energy is due to nuclear fusions.
Additionally, the reactor was opened after each tests and observation showed systematic scorching of the electrodes to such a decree that cannot possibly be explained via the sole heat produced by the electric arc due to the above indicated absence of combustion (see Figure 7.20).
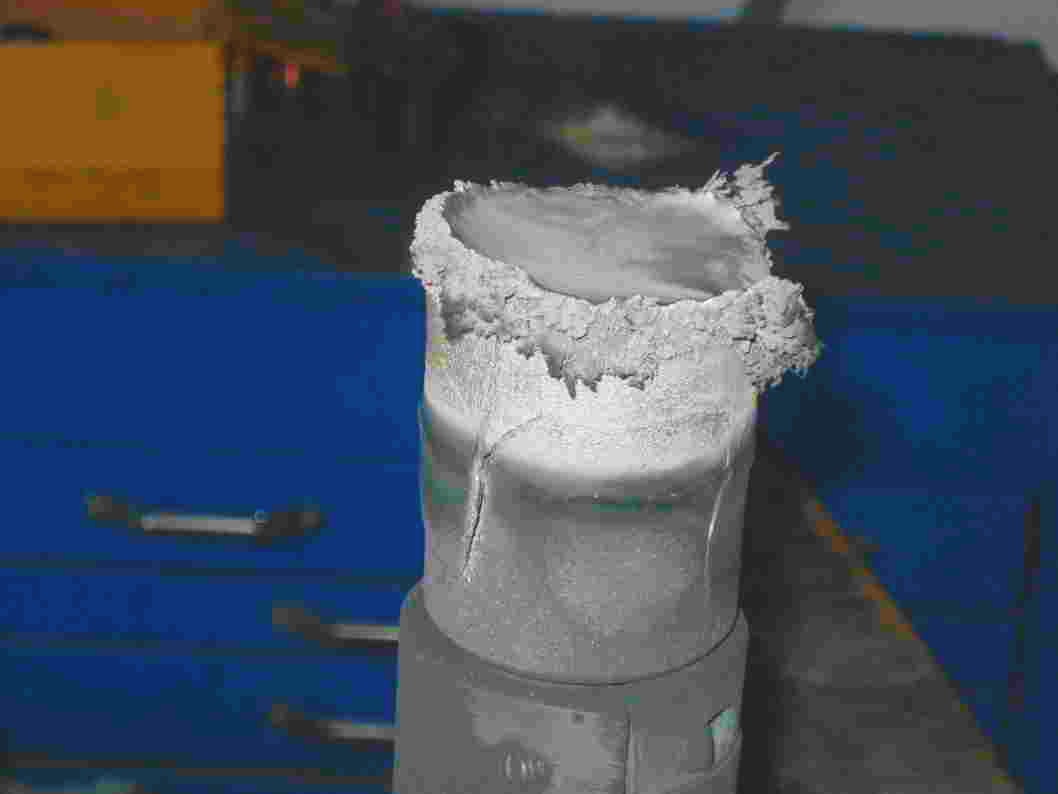
Figure 7.20. A view of the carbon electrodes following one of the tests reported below, whose whitish scorching is visual evidence of nuclear fusions since the tests dealt with a pure deuterium gas without oxygen, thus without any combustion at all, and the scorching cannot possibly have done by the arc alone.
7.3Ld. Tests with deuterium and tungsten electrodes.
In order to confirm whether or not the excess heat is specific for the combination of deuterium gas traversed by a DC arc between carbon electrodes, the author conducted systematic measurements by replacing the carbon electrodes with electrodes fabricated from commercially available tungsten and the use of a number of different gases.
It is important to report that, under the same conditions of pressure, power, duration, etc., as those of the preceding tests, the operation of the hadronic reactor with gases composed by commercially available hydrogen and air traversed by a 40 kW DC arc between tungsten electrodes produced no appreciable energy excess.
7.3Le. Tests with air and tungsten electrodes.
For comparative purposes, Santilli then conducted tests with the same equipment as above but with the reactor filled up with air and operating with tungsten electrodes. In this case Santilli measured the transition in two minutes in the cylindrical component of the reactor, from the ambient temperature of about 20o C to about 60o C, thus showing a temperature increase of about 40o C compared to the average temperature increase of the tests of the preceding section of about 127o C.
This result is, perhaps, the most important confirmation of the preceding nitrogen synthesis, because it illustrates the crucial role of carbon for the ICNF here reported.
7.3Lf. Tests with hydrogen and carbon electrodes
Santilli also conducted systematic tests to achieve ICNF via the use of the same equipment as per preceding tests, but filled up with hydrogen instead of deuterium gas and use of carbon electrodes.
Among a number of tests conducted by Santilli from January 2009 to February 2010, we report a test done on February 9, 2010 in which a vacuum was pulled out of the hadronic reactor of Figure 7.18, that was filled up with commercial grade hydrogen at 100 psi pressure; a sample of the gaseous content of the reactor was taken and market HCN1 for analysis; the reactor was then operated for two minutes under 40 Kw DC; a new sample of the gas content was taken and market HCN2 for analysis; the two samples were then shipped via FedEx Next Day Air to Oneida Research Laboratories for analysis; and some of the results are presented in Figure 7.21.
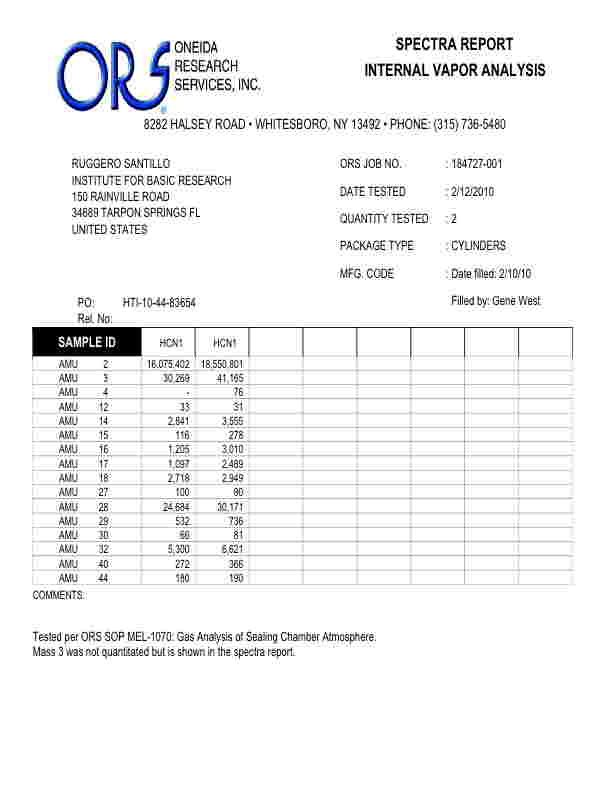
Figure 7.21. A view of the the results of the analysis of samples HCN1 and HCN2 by ORS Oneida Research Services.
The important result of this test is that under the same conditions of pressure, power, electrodes, etc., the operation with hydrogen gas produced an energy excess bigger than that with deuterium gas, since in two minutes the temperature of the reactor increased of about 50 % over the test with deuterium.
The increased energy output was predicted in the 2008 memoir due to much broader possibilities of ICNF. In fact, the analysis of Figure 7.21 shows that nitrogen (at 28 amu has indeed increased from 24,684 to 30,171 counts. However, we additionally have: the species at 4 amu increased from ND to 76 counts; the species at 14 amu increased from 2,841 to 3,555 counts; the species at 15 amu increased from 116 to 378 counts; the species at 16 amu increased from 1,205 to 2,948 counts; etc.
Consequently the measurements here reported provide experimental confirmation, not only of the synthesis of the nitrogen from hydrogen and carbon via the intermediate synthesis of the deuterium according to reactions (2.29), but also the synthesis of the oxygen, as well as other nuclear syntheses.
7.3Lg. Tests with magnegas and carbon electrodes
By still following Santilli 2010 paper, we here report that the repetition of the preceding tests via the use of the magnegas (MG) and carbon electrodes produced an energy output bigger than that of all the preceding cases.
The tests were done on November 28, 2009 by filling up the hadronic reactor of Figure 7.18 with magnegas after pulling out a vacuum and a sample bottle was taken market MG1 for analysis; the reactor was operated for one minute and a second sample bottle was taken market MG2 for analysis; the two bottles were then sent to ORS Oneida Research Laboratory for analysis via next day delivery, with results are partially reported in Figures 7.22.
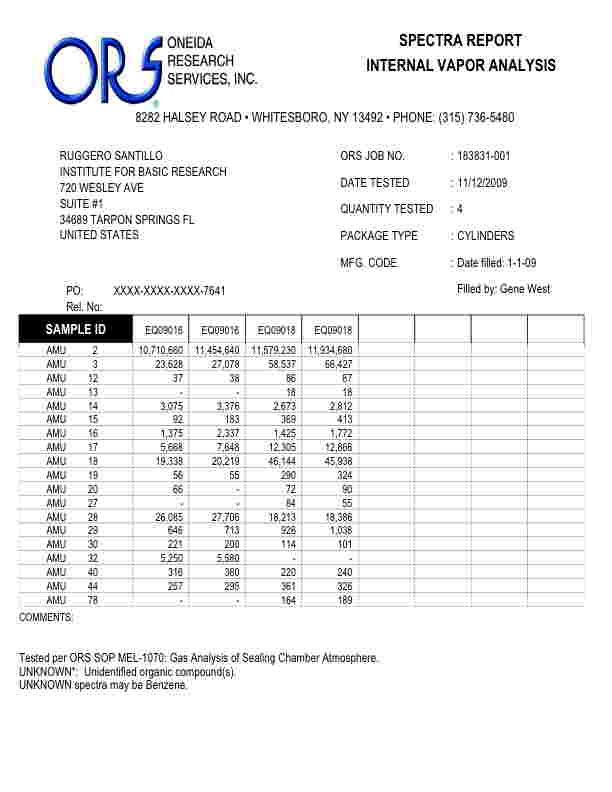
Figure 7.22. A view of the the results of the analysis of two sets of two identical samples obtained before and after the activation of the DC arc on tungsten (rather than carbon) electrodes.
On a comparative basis with preceding tests, the most significant result is that the reactor had to be stopped after only one minute of operation, rather than the two minutes of operation of the preceding tests, to avoid damage due to excess heat not being dissipated.
This result was also predicted in the 2008 memoir because the acquisition of a magnecular structure is necessary for the ICNF here considered since said structure is necessary for the exposure of nuclei and their proper spin alignment. In the preceding tests, the deuterium and hydrogen gases did not originally have any magnecular structure that, therefore, had to be created by the arc prior to possible nuclear fusions. It is then evident that the conduction of the same tests with a gas already possessing a magnecular structure must have a bigger efficiency and energy output.
The test was repeated in the same day with hydrogen, and two samples were taken market HC1 and HC2 for analyses, one before and the other after activation of the arc. The results of their analysis are combined with those for magnegas in Figures 7.22 for confirmation of the preceding results.
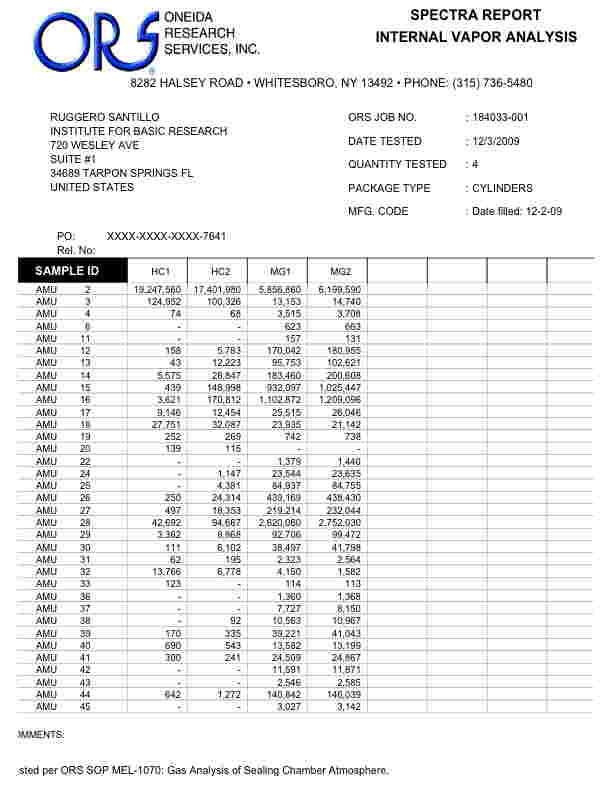
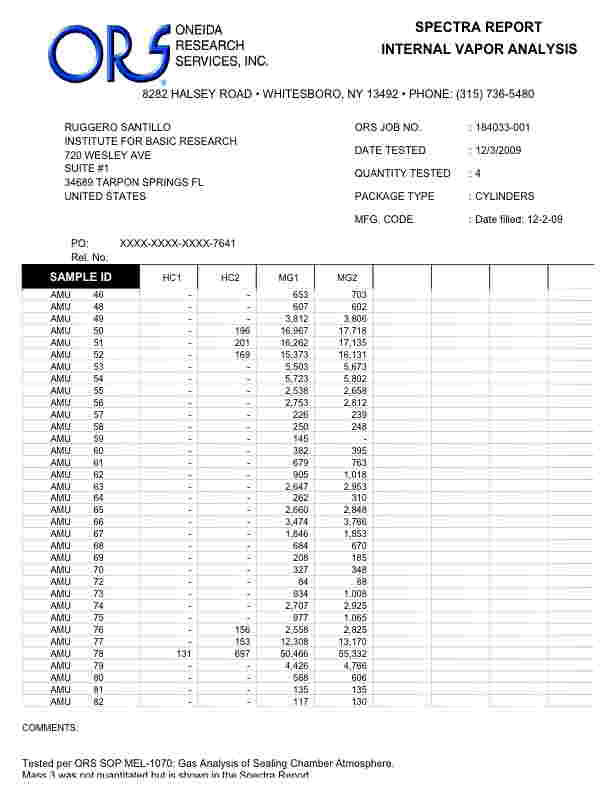
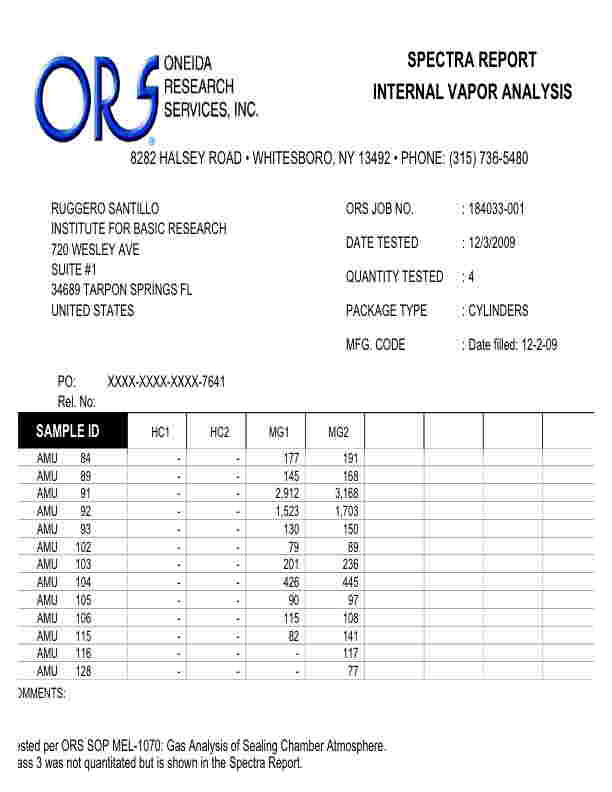
Figure 7.23. A view of the the results of the analysis of samples HC1-HC2 and MG1-MG2 by ORS Oneida Research Services.
In Figure 7.23 we report analyses on the repetition of the hydrogen tests exactly as done previously, with the sole difference that the electrodes were given by tungsten, rather than carbon rods. The tests were repeated twice in succession, resulting in the two sets of two samples of Figure 7.23, the first and third columns presenting commercial grade hydrogen with the contaminants contained in the reactor due to preceding tests, while the second and forth columns present the data on the preceding samples after activation of the arc for two minutes.
The important result is the definite increase of the species with 3 amu from 23,638 to 27,078 counts for the first set and from 58,537 to 66,427 counts for the second set of samples. Evidently, these systematic increases confirm the laboratory synthesis of the neutron from a hydrogen gas studied in Chapter 6. The neutron is then captured by the hydrogen molecule, by increasing in this way its weight to 3 amu apparently for a yet unexplored capability of electric arcs to attract polarized particles toward its symmetry axis. As indicated earlier, this synthesis is crucial for subsequent ICNF in a way similar to what is the case in the Stars.
7.3Lh. Experimental confirmation of Santilli magnecules.
Experts in quantum chemistry, but without a technical knowledge of the covering hadronic chemistry [5], may be confused by the inspection of the analytic measurements due to the presence of anomalous chemical species, i.e., species that are generally unknown, such as the species at 6 amu, the species at 19 amu (called by Santilli H3O with structure
(7.43) H3O = H2O x H = (H - O - H) x H,
and numerous others (see Chapter 4).
Anomalous species of the above type are generally interpreted in the orthodox chemical literature as being "fragments": of heavier molecules, thus maintaining the dominance of the valence bonds in the interpretation of analytic results.
However, such a conventional interpretation cannot be credibly applied to the analytic results presented by Santilli in his 2010 paper for the very simple reason that the original gas was very light, such as hydrogen, and then merely subjected to the DC arc. In fact, the latter is known not to be able to create conventional molecular species, thus leaving as the sole most plausible interpretation of the anomalous species as being composed by Santilli magnecules.
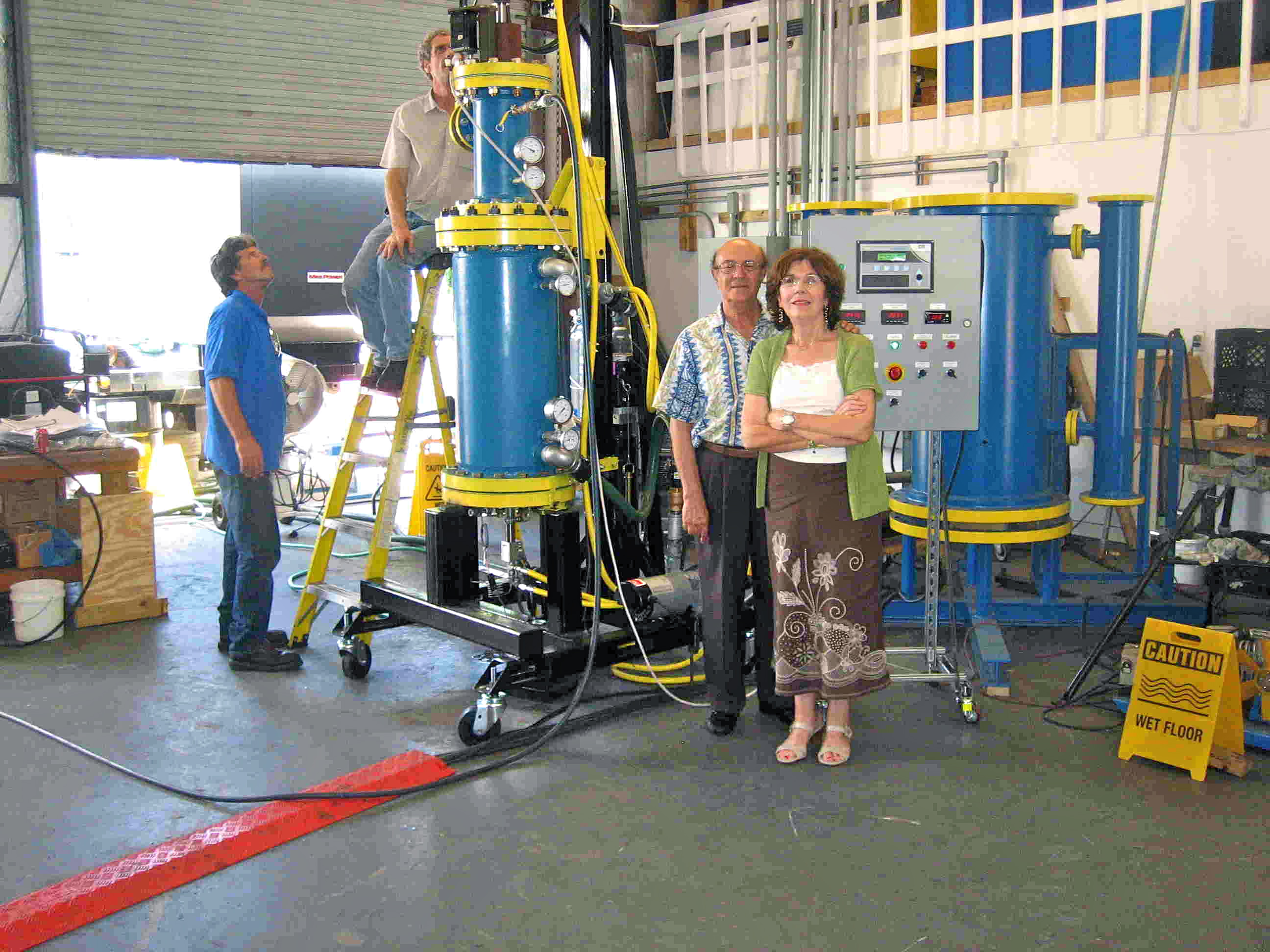
Figure 7.24. A view of Prof. and Mrs. Santilli while supervising the construction of a larger hadronic reactor for the initiation of industrial research on the ICNF (Spring 2010).
7.3Lj. Dismissal of hydrogen as hadronic fuel.
Being an environmental scientist, Santilli has spent years of research in hydrogen technologies. he does indeed supports the use of hydrogen as automotive fuel, but provided that hydrogen is produced with electrolytic processes via the use of clean renewable electric energy (such as that of solar or wind origin), and the produced oxygen is released in the atmosphere, for recombination with hydrogen at the type of combustion as a condition to maintain the current oxygen balance in our atmosphere.
However, Santilli has repeatedly expressed in his writing cautions against the widespread use of hydrogen as automotive fuel in its current form of production (via the use of non-renewable electric energy and the use of the separated oxygen) because of serious environmental problems, such as:
1) The oxygen depletion, referred to the permanent removal of breathable oxygen from our atmosphere and its conversion into H2O whose separation to restore the original oxygen balance in our atmosphere is too costly. By comparison, gasoline produces CO2 which is recycled by plants into O2. Additionally, CO2 is still contained in our atmosphere in a rather small percentage (less than 1 %), while our atmosphere contains water in large amounts (e,g.,m, up to 90 % in Florida), thus preventing any appreciable recycling of water into oxygen by plants. These are the reason mankind has survived the ongoing disproportionate use of fossil fuels. By comparison, it has been shown that, in the event the industrial revolution had occurred via the use of hydrogen as automotive fuel, humanity would have been extent because of excessive depletion of breathable oxygen below the level necessary to sustain human life.
2) The ozone depletion, referred to the permanent loss of ozone due to hydrogen seepage and its rapid raising to the ozone layer with resulting very rapid reaction
(7.44) H2>2 + O3 → H2>2O + O2.
3) The need of liquefy hydrogen and maintain it as such,, which is necessary for hydrogen to have any appreciable automotive range, with serious risks in the event of a malfunction of the cooling system;
4) The pollution created by the current means for hydrogen production, such as in the reformation of fossil fuel such as methane CH2>4; releasing vast amounts of green house gases CO2>2;
5) The well known problem called embrittlement that render steel pressure bottle prone to explosion, and other problems.
Additionally, Santilli strongly discourages the use of hydrogen as hadronic fuel, that is, as fuels for his ICNF for the evident reason that its use implies the necessary synthesis of neutrons as studied in detail in the preceding section.
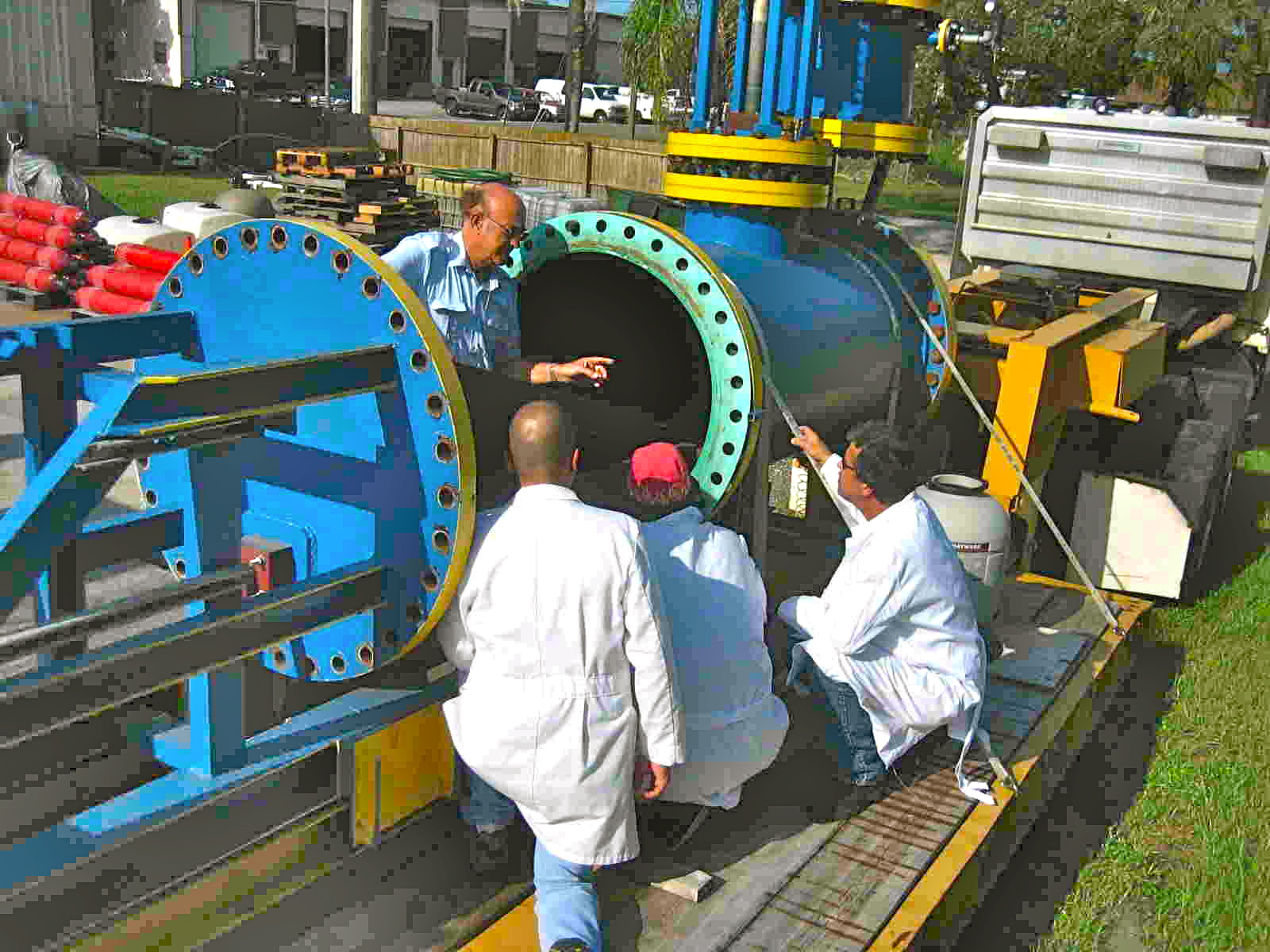
Figure 7.25. A view of Prof. Santilli and IBR technicians while discussing the construction of a large industrial hadronic reactor 9Summer 2010).
In conclusions, Santilli recommends in his 2010 paper the selection of any desired hadronic fuel under the indicated priority of not releasing harmful radiations and/or radioactive wastes. These conditions inevitably focus the attention on the hadronic fuels selected by Santilli, namely, the ICNF of light, natural and stable elements into light, natural and stable element.
7.3M. Independent verification of Santilli's nitrogen synthesis without harmful radiation or waste
7.3Ma. foreword We now review the paper
Verification of Santilli's intermediate nuclear fusions without harmful radiation
and the production of magnecular clusters
R. Brenna, T. Kuliczkowski, L. Ying
Princeton Gamma-Tech Instruments (PGTI), Princeton, NJ
In press at New Advances in Physics, in press (2010),
The above identified experimentalists under the leadership of Leong Ying spent one week in mid March 2010at the laboratory of The Institute for Basic Research in Tarpon Springs, Florida, for the specific purpose of independently confirming or denying Santilli's measurements presented in the preceding section.
For this purpose, said experiemntraliusts had to use exactly the same experimental set up as used by Santill so as to avoid the risk of running the same experiments. Santilli did not participate to the measurements, but the IBR technicians G. West, M. Rodriguez, J. Alban and others assisted L. Ying and his associates in the exact repetitiopn of the synthesis of nitrogen from a deuterium gas and carbon electrodes as outlined in Section 7.3L.
Following expensive and repeated measurements, the experimentalists confirm both the experimental detection of magnecular clusters as well as the nitrogen synthesis by reaching the following conclusion:
The results taken from the experimental runs conducted on the hadronic reactor indicates some form of exothermal reaction taking place that produced clusters of higher mass components. Since chemical reactions and combustion cannot have occurred in a pure deuterium environment, the conclusion leads to an indication of the process described as Intermediate Controlled Nuclear Fusion without harmful radiations.
7.3Mb. Deuterium-carbon fusion
The above important verification can be summarized as follows. The objective was the study of Santilli's ICNF of deuterium and carbon by the ICNF process to form nitrogen, which can be described using hadronic mechanics with the following reaction in the symbols identified above
(7.45) TR + H(2, 1, 1+, 2.0141) + C (12, 6, 0+, 12.0000) →
→ N (14, 7, 1+, 14.0030) + 0.0111amu (= 10.339MeV),
The trigger (TR) mechanism to initiate the reaction process is the electric arc that polarizes the carbon and hydrogen atoms to form magnecular clusters. On the atomic distances between the axially coupled atoms, the extremely strong magnetic fields generated by the arc toroidally deform the atomic orbitals and thereby exposing the nuclei from their electronic clouds. The close proximity of the bare nuclei leads to the nuclear fusion with the generation of excess heat.
The hadronic reactor was pressurized with pure deuterium gas by first evacuating with a mechanical vacuum pump the chamber and then backfilling with the gas from a supply bottle. Gas samples were taken before and after each initiated reaction, and sent to an independent laboratory [4] for spectra vapor analysis.
Each experimental run was started close to ambient temperature of nominally 25o C, with the electric arc powered for 2 minutes. The wattmeter measured an average power consumption of 1550 W.hr, which equates to an energy input of 5.4 MJ. A total of 3 runs were performed at varying starting pressures of 100, 75 and 50 psi.
For the 100psi tests, gas samples before (A) and after (B) was taken. The reactor chamber was then purged and refilled with pure deuterium, and a gas sample (C) was taken at a starting pressure of 75psi. After the reaction process at 75psi, a gas sample (D) was extracted. The reactor was then allowed to cool back to ambient and the pressure reduced to 50psi for another reaction, and a final gas sample (E) taken.
7.3Mc. Gas spectra analysis
Deuterium is non-combustible, and there were also negligible amount of oxygen contained in the hadronic reactor for any other combustion processes to have occurred. Hence if there were no hadronic chemistry or fusion processes taking place then we would expect to observe similar vapor spectra for the samples taken before and after initiation by the electric arc. The analyzed mass spectra for the 5 gas samples, the reported values in parts-per-million (ppm) by volume were accurately reported in the table below.
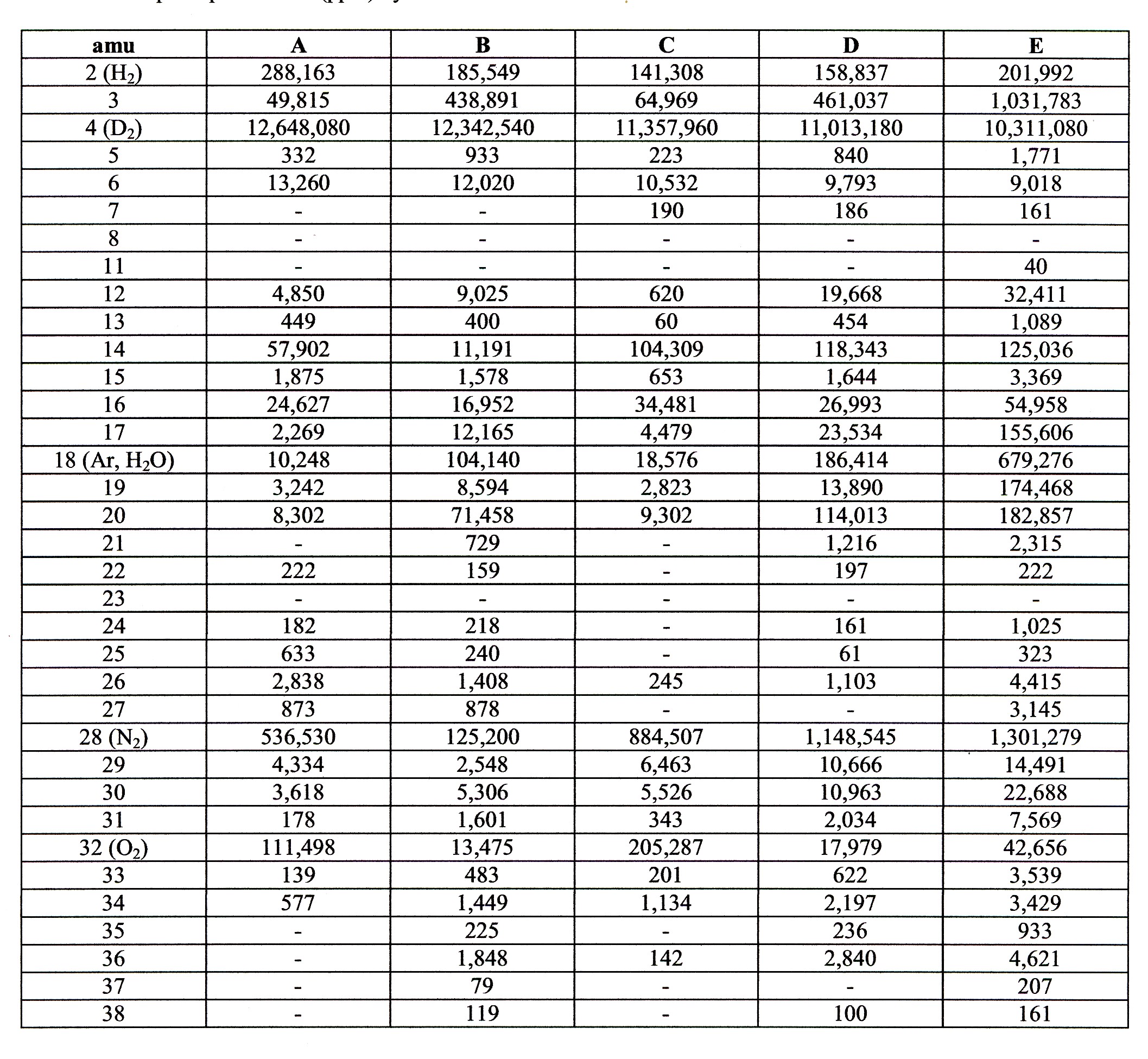
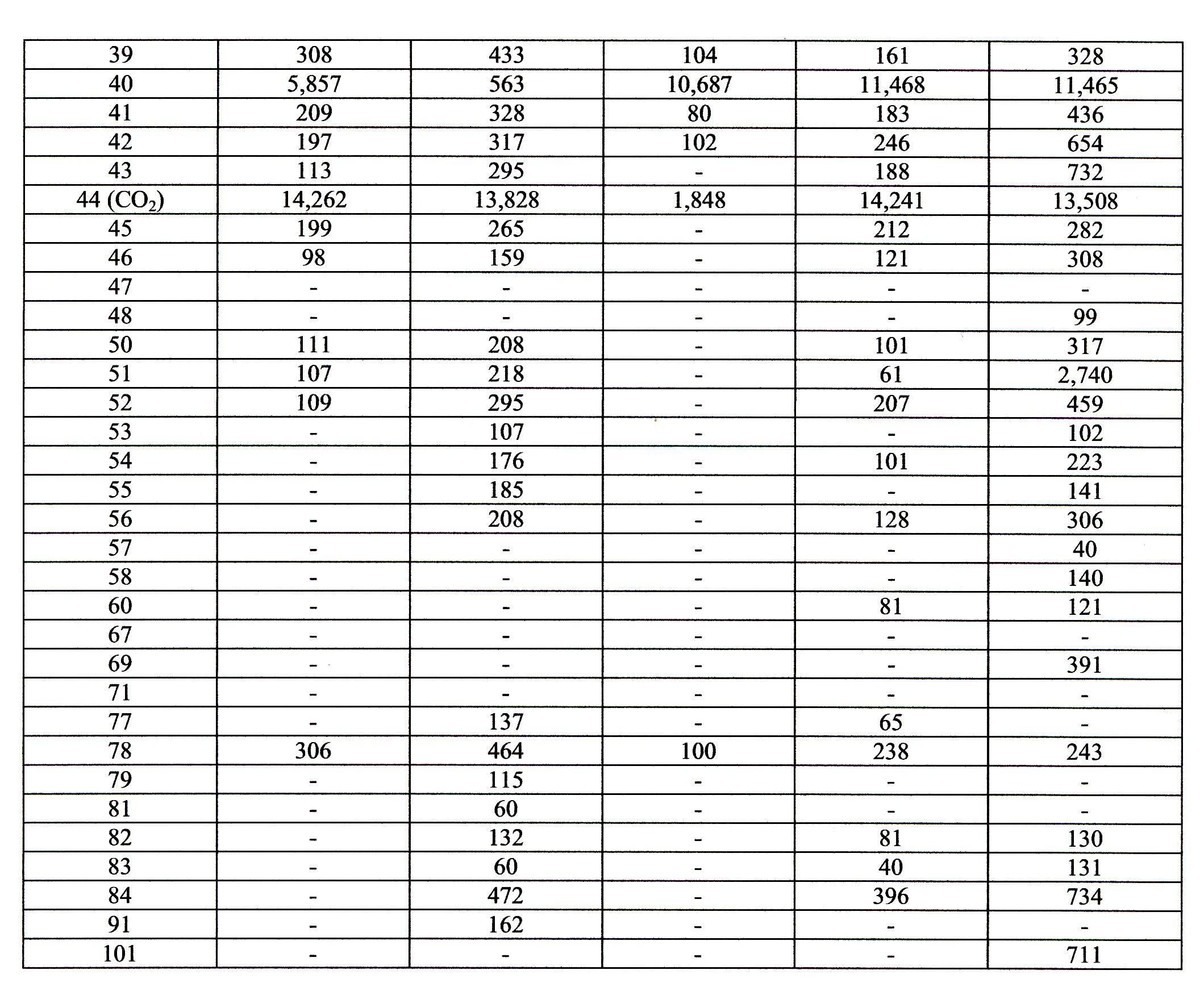
Figure 7.26. A view of the the data collected by L. Yiung and associates on the five samples of tested gases.
The spectral analysis indicates a reduction in the amount of deuterium following each reaction. At 100 psi (A → B) the decrease was approximately 2.5%, and at 75 psi (C &rarrl D) it was 3%. The decrease in the amount of nitrogen in the 100 psi data can be misleading, since the evolved nitrogen can be trapped in clustered magnecules as indicated by the existence of higher mass entities in the spectral data following all the reactions. These previously unknown higher mass magnecules are further evidence of the hadronic chemistry taking place.
7.3Md. Elemental microanalysis
Samples of deposits on the surface of the graphite electrodes were removed for material characterization in a Scanning Electron Microscope (SEM) using an Energy Dispersive Spectroscopy (EDS) x-ray detector. The detector is a liquid-nitrogen cooled lithium-drifted silicon crystal biased to operate as a semiconductor junction. X-rays liberate electron-hole pairs in the junction, and the amount of charge collected is proportional to the x-ray energies. The electron beam striking the samples generates electronic excitation, and it is the decay of these electronic shells that emits the characteristic x-ray energies unique to each element.
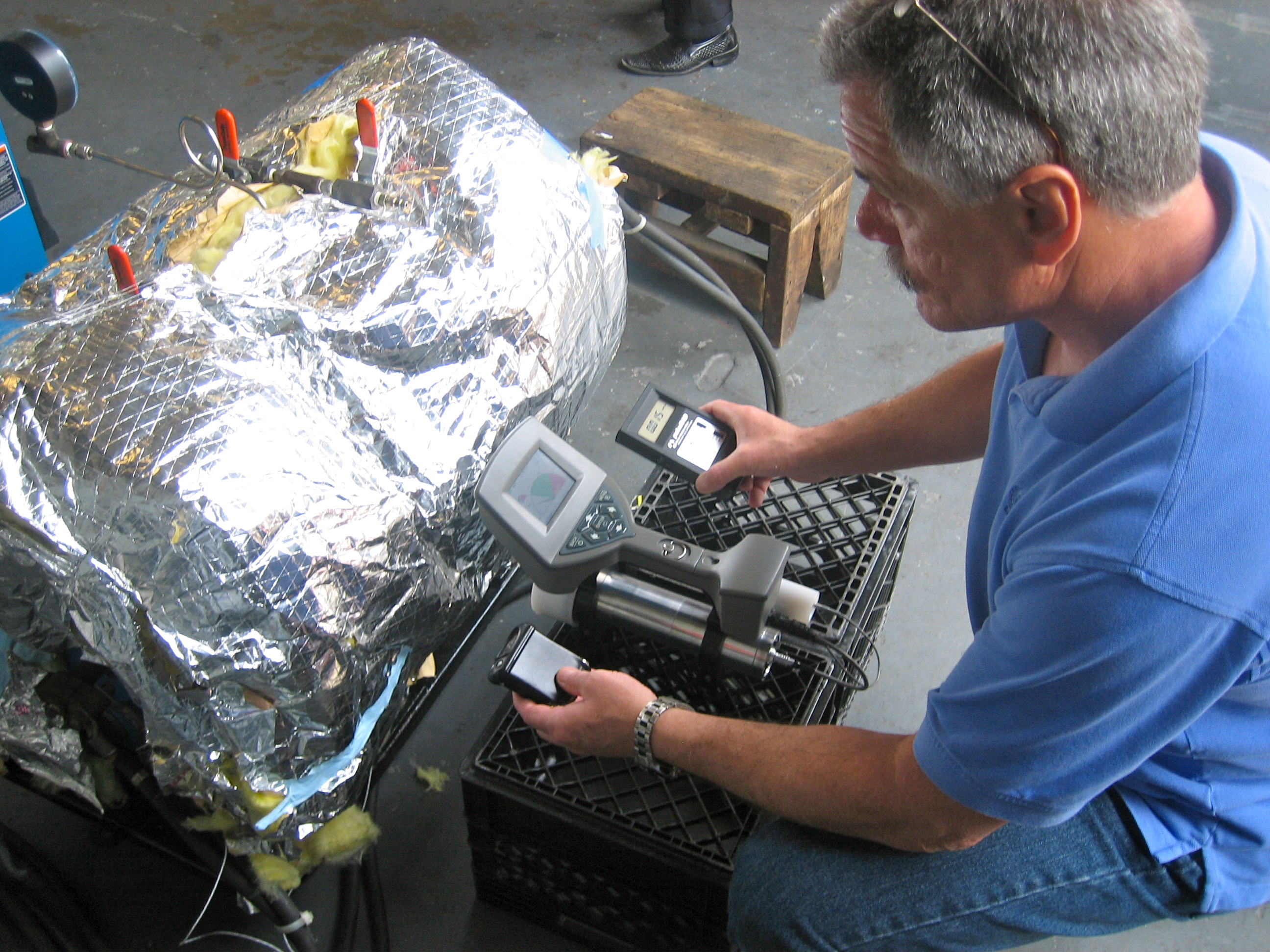
Figure 7.27. A view of T. Kuliczkowski from PGTI) during one of the several measurements of lack of any harmful radiation with three different detectors.
The EDS detector is a PGT's model LS10133 mounted to an ISI Super IIIA SEM. The samples were epoxied to a holder placed directly in line with the electron beam. The long vacuum insulated endcap housing the Si(Li) crystal is inserted into the SEM chamber in close proximity to the sample. Fluorescence x-rays scattering off the target sample and entering the endcap through a thin-walled polymer window are identified by the EDS detector system.
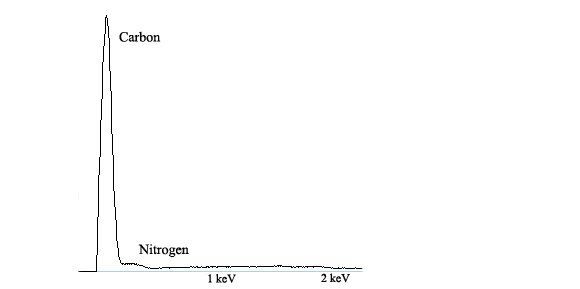
Figure 7.28. Elemental spectra of deposits on graphite electrode
The elemental microanalysis spectra taken on the surface deposits of the graphite electrodes show a prominent x-ray peak at 277 eV (carbon K?). There is a small adjacent peak at 392eV, which is the nitrogen Kα x-ray that is noticeable above the general background level. Since the SEM chamber is under vacuum, then the detected nitrogen must exist in some non-gaseous form, possibly within clustered magnecules.
7.3Me. Thermal Analysis
Platinum resistive temperature sensors were securely fastened to the surfaces of the steel chamber's central tube and one of the endplates. Temperature readings were noted down each minute after the electric arc was powered up to produce a thermal profile of the hadronic reactor. A thermal Finite Element Analysis (FEA) was simulated [7] for the reactor to estimate the expected temperature rise if the only source of heat came from the electric arc. Comparison curves of the measured thermal profiles against the FEA computed values at 5MJ, 5.5MJ and 6MJ energy inputs are shown below.
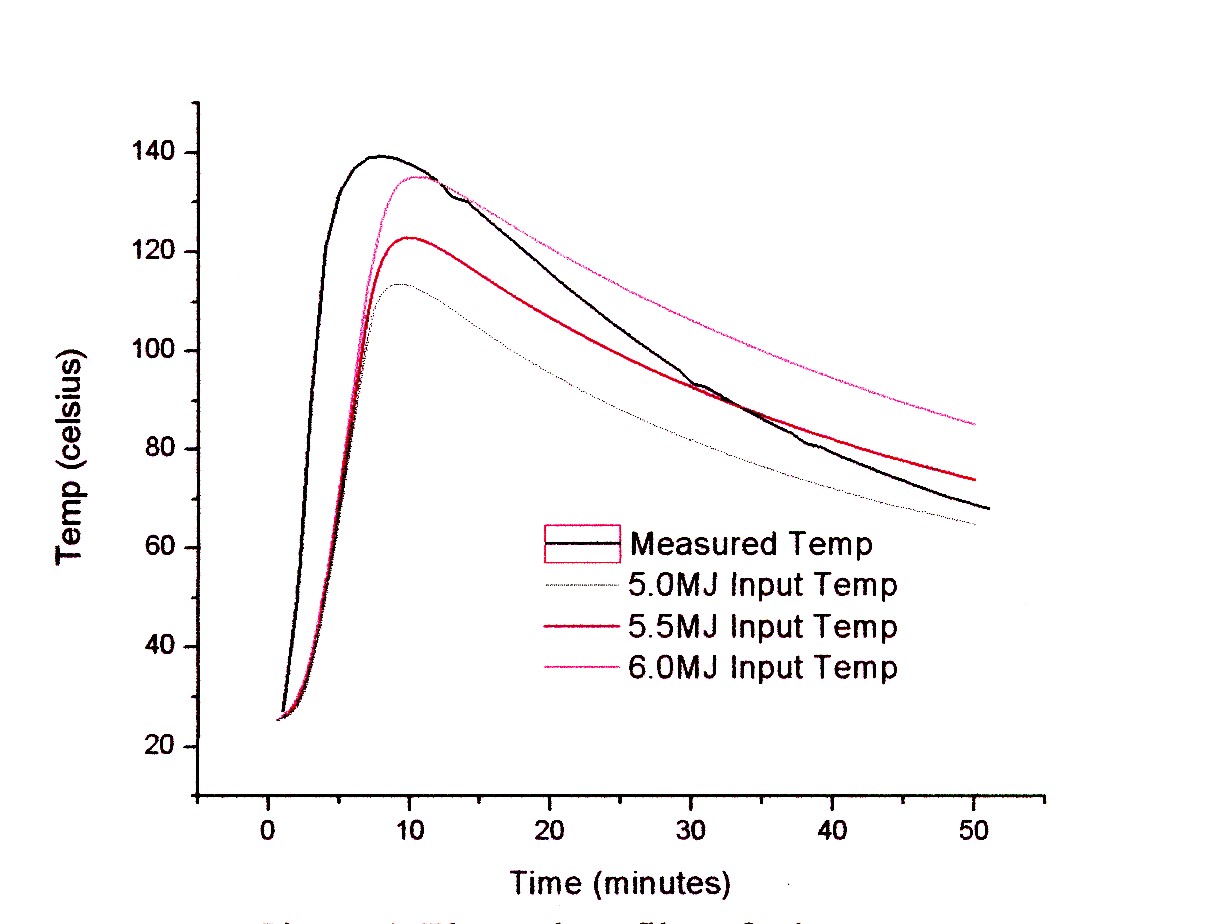
Figure 7.29. Thermal profiles of tube
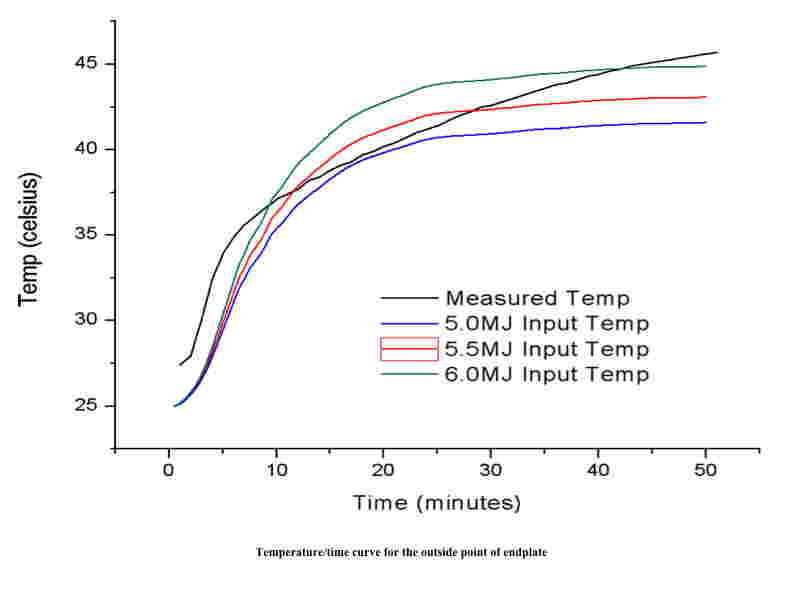
Figure 7.30. Thermal profiles of endplate
The data indicates the generated excess heat Eheat of approximately 0.5MJ above the total injected energy input of 5.4MJ from the electric arc. From equation (1) we note that each reaction releases around 10MeV of fusion energy, hence if we assume all the excess heat is through the ICNF process, then this is equivalent to the generation of roughly 1018 or a micro-mole of fusion products.
7.3Mf. Radiation Analysis
The SAM940 sodium iodide scintillator detector is self-calibrating at the potassium (40K) energy of 1.461MeV. The helium (3He) proportional counter was factory calibrated against a californium (252 Cf) neutron source. For safety and security reasons the source is embedded in wax and locked inside a steel vault. Opening the vault door and placing the SAM940 instrument approximately a meter from the source, we were able to detect average neutron levels of 0.8 counts per second (cps). With the vault door closed and the instrument removed from the vicinity, the background levels fell to less than 0.03 cps.
Compared to normal background levels there were no emitted gamma-rays or neutrons detected emanating from the hadronic reactor during the fusion process occurring within the chamber.
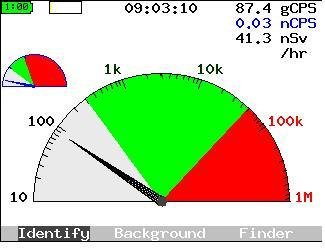
Figure 7.27. SAM940 Gamma and neutron detection
7.3Mg. Conclusions.
In conclusion, the 2010 paper by L. Ying and his collaborators confirms all discoveries presented by Santilli in his 2010 paper, namely:
1) Due to the lack of any possible combustion in a metal chamber filled up with pure deuterium gas traversed by a DC arc between carbon electrodes, the excess energy detected by the experimentalists over the energy of the DC arc is necessarily due to nuclear fusions. Inspection of chemical analyses before and after the tests as well as examination of the case, reveal that the sole possible fusion is that first achieved by Santilli from deuterium and carbon.
2) Systematic measurements conducted with various detectors have confirmed that no harmful radiation of any type was detected in any of the tests outside the hadronic reactor, thus confirming that said nitrogen synthesis occurs without the emission of neutron or other harmful radiation. Additional inspections of the interior of the hadronic reactor following the tests confirmed Santilli's finding that said nitrogen synthesis is achieved without any release of harmful waste. In any case, a study of the case establishes the lack of energy necessary for the fission of the carbon and/or deuterium nuclei as a pre-requisite for the emission of harmful radiation, as a result of which either the deuterium and carbon nuclei fuse into the nitrogen nucleus, or they do not, without any possibility of releasing harmful radiation or waste.
3) Examination of chemical analyses of the deuterium gas before and after being traversed by a DC arc establishes the creation in the latter case of new heavy chemical species detectable all the way to 400 amu, a number of which detected in macroscopic percentages, which new species cannot possibly exist in a pure deuterium gas. This evidence disproves in a final way the widespread opinion that the new species are "fragments" of heavier molecules, since the latter did not exist in the original deuterium gas. The same evidence dismisses the possibility that the new species have a valence bond since DC arcs notoriously break down valence bonds and cannot possibly create any. Consequently, the independent evidence gathered by the three nuclear physicists from Princeton, NJ, provides experimental confirmation of the existence of the new chemical species of Santilli magnecules.
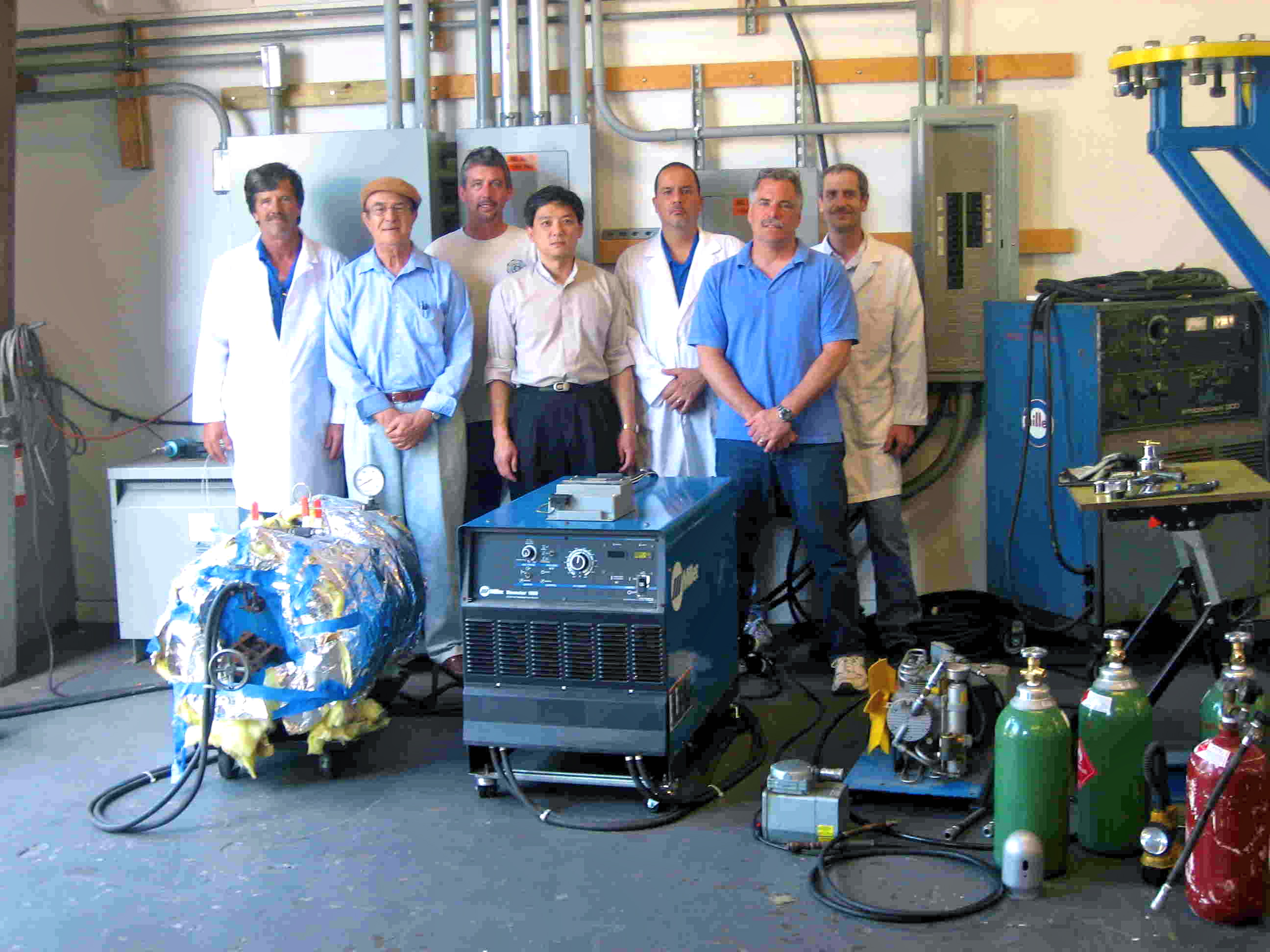
Figure 7.28. A view of the participants in the verification of the nitrogen synthesis from carbon and deuterium, showing from the left: G. West (IBR), R. M. Santilli (IBR), R. Brenna (PGTI), L. Ying (PGTI), M. Rodriguez (IBR), T. Kuliczkowski (PGTI), and C. Lynch (IBR). The picture also shows the used equipment consisting from the left: the hadronic reactor of Figure 7.18 with proper thermal insulation; the Miller Dimension 1000 AC-DC converter with accurate wattmeters in its top; the vacuum pump; various deuterium pressure bottles; and a number of radiation detectors, temperature sensors and other instruments.
To be competed with additional experimental verifications of the synthesis of the Nitrogen from Carbon and Deuterium plus the synthesis of the Silica from Oxygen and Carbon and other nuclear syntheses without radiations.
7.4. CLEAN ENERGY FROM THE STIMULATED DECAY OF THE NEUTRON.
7.4A. Introduction
In this section we introduce a third class of new hadronic energies, those of particle type in the sense that they originate in the structure of individual composite particles, rather than in their collection. The new energy was called hadronic because essentially dependent for its prediction and quantitative treatment of hadronic mathematics, mechanics and chemistry.
This third class of new energies was introduced for the first time in the paper that initiated the content of this chapter, hereinafter referred as the 1994 paper,
Hadronic energy
R.M. Santilli, Hadronic Journal, Vol. 17, 311 (1994)
which remains to this day the most comprehensive and authoritative treatment in the field.
Santilli's last study of the new hadronic energy of particle type can be found in the 1999 monograph
The Physics of New Clean Energies and Fuels According to
Hadronic Mechanics,
R.M. Santilli,
Special issue of the Journal of New Energy, 318 pages (1999).
In this section, we shall follow almost ad litteram the excellent review by Jerdsay Kadeisvili of2008
The Rutherford-Santilli neutron
J. V. Kadeisvili,
Hadronic J. Vol. 31, 1-114 (2008)
Additional; contributions will be quoted when needed.
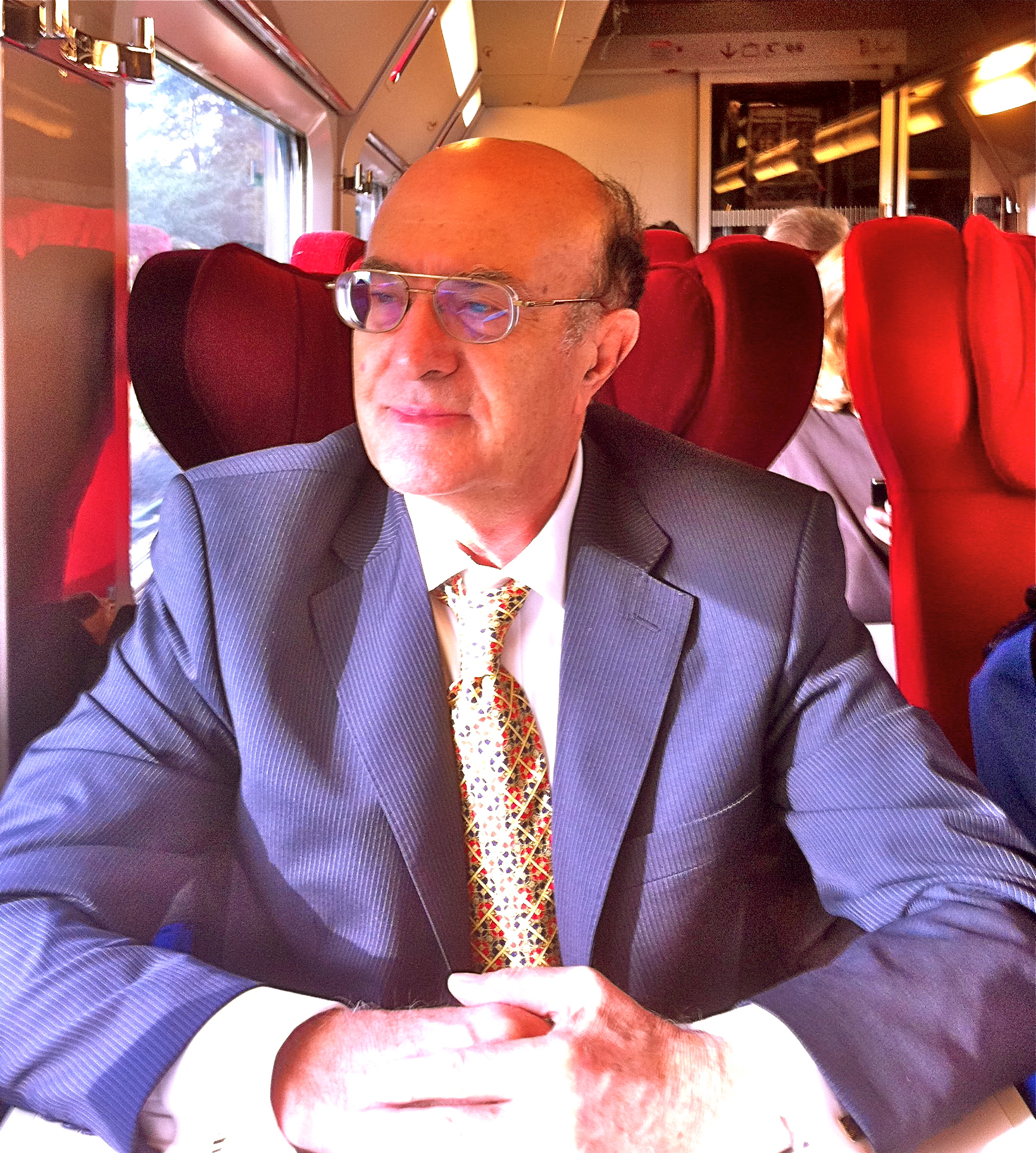
Figure 7.33. A picture of Prof. Santilli on September 25, 2010, at age 76, taken by his son Ermanno on a "Train de Grand Vitesse" in Europe while heading to deliver a lecture at the WETSUS Institute in The Netherlands (a world leader for studies on water) on the need to verify experimentally the inapplicability of special relativity within physical media such as water (see Chapter 5), due to very large scientific, social and environmental implications, including the capability to predict, basically new, much needed new clean energies studied in this chapter.
7.4B. The stimulated decay of the neutron
Santilli has repeatedly stressed in his writings that the neutron is an unlimited possible source of energy because it can decay via the release of a highly energetic electron easily trapped with a metal shield, plus the innocuous neutrino, if it exists.
Following his research on the synthesis of the neutron outlined in the preceding chapter, Santilli conducted comprehensive studies on the stimulated decay of the neutron because of the possibility of producing a new form of clean energy he called hadronic energy from the methods of its conception and treatment.
Whether successful or not, these studies are the very first and only studies known to the author on possible practical applications of hadron physics. In fact, the theory of electromagnetic interactions produced historical, well known applications while, by comparison, prior to Santilli's 1994 paper the theory of strong interactions had produced no practical application whatsoever, not even remote or conceivable.
As it is well known, the neutron is naturally unstable with a meanlife when isolated of about 15 m and with a variable meanlife ranging from a few seconds, when member of certain nuclei, all the way to full stability, when member of other nuclei. Hence, it is quite plausible to expect that the neutron admits one or more triggers (TR) under which we have the stimulated decay, [24,43]
(7.46) TR + n → p+ + β-,
where β- is conventionally interpreted, e.g., as having spin zero for the conservation law of the angular momentum when the trigger has also spin zero (the case with spin 1 will be indicated when needed). In particular, β- can be interpreted either as an electron and a neutrino or as an electron and an antietherino with opposing spin 1/2. This difference is irrelevant for the stimulated decay of the neutron and, consequently, it will be ignored hereon.
Stimulated decay (7.46) is strictly prohibited by quantum mechanics at large and by the standard model in particular. Under the belief that quarks are the actual physical constituents of hadrons, there is no possibility to stimulate the decay of the neutron, and this illustrates the social, let alone scientific implications of the belief without serious scrutiny that quarks are physical particles in our spacetime.
However, possibility (7.46) is clearly predicted and quantitatively treated by the covering hadronic mechanics. In fact, hadronic mechanics predicts a variety of possible realizations of the trigger, including triggers acting in the interior of individual neutrons or of nuclei, nuclei, including the possible disruption of the nonpotential component of the nuclear force.
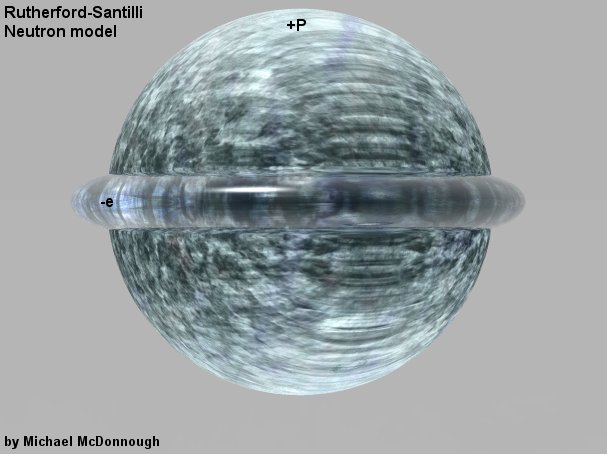
Figure 7.34. An illustration of the support by the industry of research on new clean energies requiring suitable coverings of 20th century doctrines, depicting the conception by Michael McDonnough, President of BetaVoltaic, Inc., of the "Rutherford-Santilli neutron" that is at the foundation of its possible stimulated decay and related new clean energies.
It should be indicated that, despite vast and widespread skepticism in academia still persisting at this writing (fall 2010), the stimulated decay of the neutron has received significant research funds and support from the industry due to the well known need for new clean energies as the only way for containing increasingly alarming climactic events,
7.4C. Neutron stimulated decay via photons with resonating frequency
In Chapter 6, we have reviewed Santilli's synthesis of the neutron from a proton and an electron in mutated isotopic forms. In particular, we have shown that the isoelectron in the neutron structure is essentially free under MeV energy ranges, because the binding force is not derivable from a potential, thus carrying no energy. We do have an attractive Coulomb bond derivable from a potential, thus having a negative binding energy, but its (absolute) value is small in MeV unit, thus belonging to refinements not considered here.
Additionally, we have shown that, in the transition from motion in empty space to motion within the hyperdense medium inside the proton, the electron experiences an alteration of its rest energy, called isorenormalization, of purely geometrical character due to the mutation of the Minkowskian spacetime caused by hyperdense media, geometric deviations also visible in the variation of the speed of light within physical media and numerous other events.
Therefore, in his 1994 paper Santilli proposed, apparently for the first time that the neutron can be stimulated to decay via the use of a photon γr with a resonating energy (frequency) "r" that is an integer multiple or submultiple of the isorenormalized energy of the isoelectron,
(7.47a) γr + n → p+ + β-,
(7.47b) γr = n x 1.294 MeV, or n x 3.129 x 1020 Hz,
(7.47c) n = 1, 2, 3, 4, ...; or n = 1/2, 1/3, 1/4, ...
where the β carries 0.782 MeV of usable energy that is the main target for practical uses.
Jointly, Santilli suggests to consider the natural characteristic frequency of the electron in vacuum as a potential resonating photon, namely
(7.48a) γr' = n x 0.511 MeV, or n x 1.236 x 1020 Hz,
(7.48) n = 1, 2, 3, ...; n = 1/2, 1/3, 1/4, ...
Reactions (7.47) or (7.48) are not referred to an isolated neutron in vacuum, but to a neutron when member of a nuclear structure. Hence, in conventional nuclear symbols A, Z, J, amu, the reaction under consideration is written
(7.49) γr(0, 0, 1) + N(A, Z, J) → N(A, Z+1, J+1) + β-(0, -1, 0),
under the verification of all nuclear laws and superselection rules, including the conservation of the energy, charge, angular momentum, parity, etc. Additionally, the resonating frequency has to be adjusted for nuclear binding forces solely of proved potential origin [24].
The mechanism for stimulated decay (7.49) is elementary. The resonating photon hitting a nucleus is expected to excite the isoelectron inside a neutron irrespective of whether the photon penetrates or not inside the neutron. Once excited, there is no possibility for the isoelectron other than that of leaving the neutron structure, thus causing its stimulated decay.
This is due to the fact that hadronic mechanics predicts one and only one energy level for the proton and the electron in conditions of total mutual immersion, the neutron. The range of hadronic mechanics is essentially given by the radius of the neutron (1 fm). Once excited, the isoelectron has no other possibility than that of exiting the proton and reassuming its conventional quantum features when moving in vacuum.
Numerous additional triggers are predicted by hadronic mechanics. Another one Santilli Santilli has been authorized to disclose by his investors is the use of photons with a wavelength equal to the neutron size. In this case, we have the excitation of the neutron as a whole, rather than the isoelectron in its interior, but the predicted result is always the stimulated decay.
As also shown in Chapter 6, see, once the neutron is established as being a bound state of a proton and a (mutated) electron, nuclei result as being new bound states of protons and electrons, the old interpretation as bound states of protons and neutron being only a first approximation. Under these new vistas, stimulated decay (7.49) is quite plausible because applicable, for instance, to the isoelectron during exchanges between protons.
Since in practical applications nuclei will not be hit by individual resonating photons, by by their coherent beam, Santilli also proposed the study of multiple stimulated decays of peripheral neutrons in a nucleus
(7.50) n γr(0, 0, 1) + N(A, Z, J, 0) → N(A, Z+n, J + m) + n β-(0, -n, 0),
where n = 1, 2, 3, ... and the value of m depends on possible polarizations.
Specific examples were proposed in the 1994 paper, among which we recall the use of the isotopes Li(6, 3, -1), Zn(70, 30, 0) S(32, 16, 0) and others (see, for details, the 1999 monograph).
7.4D. Hadronic energy of particle type
Nowadays, there are various forms of hadronic energies under study by the industry. The form that has been disclosed at this writing is based on double beta decays of the type
(7.51a) γr(0, 0, 1) + N(A, Z, J) → N(A, Z+1, J+1) + β-(0, -1, 0),
(7.51b) N(A, Z+1, J+1) → N(A, Z + 2, J+) + β-(0, -1, 0),
where the first reaction is stimulated and the second is spontaneous.
The original isotope is selected in such a way to meet the following conditions:
1) Admits the stimulated decay of at least one of its peripheral neutrons via one photon with a resonating frequency verifying all conservation laws of the energy, angular momentum, etc.;
2) The new nucleus admits a spontaneous beta decay so that with one resonating photon we have the production of two electrons whose kinetic energy is trapped with a metal shield to produce heat;
3) The original isotope is metallic so that, following the emission of two electrons, it acquires an electric charge suitable for the production of a DC current between metallic the isotope and the metallic shield;
4) The energy balance is positive; and, last but not least
5) The initial and final isotopes are light, natural and stable elements so as to have a new energy that is clean in the sense of producing no harmful radiations (since the electrons can be easily trapped with a thin metal shield), and leave no radioactive waste.
When the original isotope meets the above requirements, it is called hadronic fuel, and the equipment used for its production is called hadronic reactor. It should be stressed that the word "hadronic" here is not intended to strong interactions, but to the use of hadronic mechanics.
7.4E. Hadronic Energies via double beta decays
As a result of comprehensive studies, Santilli has indicated that most nuclei do not admit stimulated double decays. However, there exists indeed a class of nuclei admitting indeed the double beta decay, as it is the case for Mo(100, 42, 0), However, as studied, for instance, in the experimental paper
A. Garcia et al, Phys. Rev. C Vol. 47, page 2910 (1993)
the rate of double decay is very small, thus having no practical value.
Santilli has shown that the above studies are based on the ?\conventional assumption that neutrons are the final constituents of nuclei (jointly with protons). When neutrons are assumed as a bound state of a proton and an isoelectron, hadronic mechanics does indeed predicts an industrially meaningful rate of double beta decay of the MO(100, 42, 0).
Additionally, Santilli points out that the peak in the rate of double beta decay occurs, specifically, at the resonating frequency that has never been tested under quantum mechanics. In different words, the calculations the double beta decays made via quantum and hadronic mechanics coincide everywhere except for a peak at the resonating amplitude solely admitted by hadronic mechanics that deserves serious study due to the environmental,. let alone scientific implications.
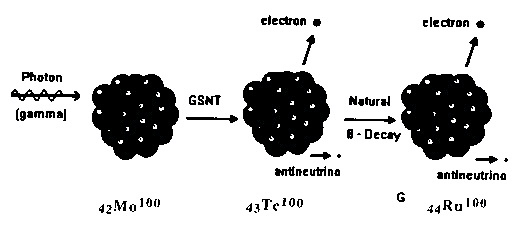
Figure 7.35. A schematic view of the example of hadronic fuel proposed by Santilli in his 1994 paper, the isotope of molybdenum Mo(100, 42, 0). Note that all other Molybdenum isotopes were proved not to admit a stimulated beta decay. Note also that quantum mechanics does predict the double beta decay, but with very small amplitude, thus being of no practical value. Stimulated double decay in appreciable percentage are solely predicted by hadronic mechanics and specifically when using photons with a specific resonating frequency never studied via quantum mechanics.
Under the above clarifications, Santilli studied double beta decay as described by hadronic mechanics
(7.53a) γr(0, 0, 1) + Mo(100, 42, 0) → Tc(100, 43, 1) + β-(0, -1, 0),
(7.53b)Tc(100, 43, 1) → Ru(100, 44, 0) + β-(0, -1, 1),
where, by using the data from the Table of Nuclides http://atom.kaeri.re.kr/, we have:
a) Mo(100, 42, 0) is naturally stable with mass 99.9074771 amu;
b) Tc(100, 43) has mass 99.9076576 amu and is naturally unstable with spontaneous decay into Ru(100, 44, 0) and half life of 15.8 s;
c) Ru(100, 44) is naturally stable with mass 99.9042197 amu.
As one can see, the mass of Mo(100, 42, 0) is smaller than that of Tc(100, 43, 1). yet, the conservation of the energy can be verified with a resonating frequency of 0.16803~ MeV (obtained for n = 1/7).
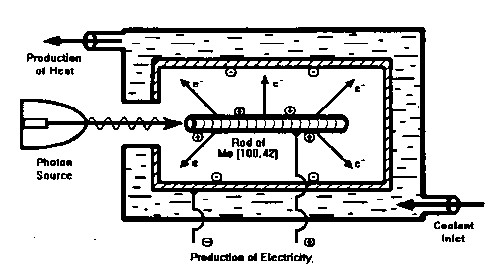
Figure 7.36. A schematic view of the hadronic reactor proposed in the 1994 paper consisting of: a coherent beam of resonating photons hits a bar of Mo(100, 42) with the stimulated transmutation into Tc(100, 43) with the emission of a first highly energetic electron, followed by the spontaneous decay of Tc(100, 43) into Ru(100, 44) with the emission of a second highly energetic electron. The electrons are captured by a metal shield that absorbs also the energy corresponding to the decrease in mass from Mo(100, 42) to Ru(100, 44). Additionally, the difference in potential between Mo(100, 42, 0) and the shield produces a DC current.
But the mass of the original isotope is bigger than that of the final isotope for a value much bigger than that of the resonating photon. with usable hadronic energy (HE) power nuclear reaction
(7.54) HE = M(100, 42) - M(100, 44) - E(γ) - 2 x E(e) =
= 3.034 - 0.184 - 1.022 MeV = 1.828 MeV,
where Santilli subtracts the conventional rest energy of the two electrons because not usable as a source of energy in this case.
The predicted hadronic energy in this case is two-fold, because we first have the production of heat acquired by the shield capturing the electrons and, jointly, we have the production of a DC electric current between the metal isotope Mo(100, 42, 0) acquiring a positive charge due to the loss of two electrons per reaction, and the metal shield acquiring two negative charges, by keeping into account that each resonating photon produces two electrons.
To appraise the usable energy, let us recall again, for convenience, the following units and their conversions
(7.55) 1 amu = 931.494 MeV; 1 MeV = 1.602 x 10-13 J = 4.45 x 10-17 Wh =
= 1.511 x 10-16 BTU; 1 Wh = 3.397 BTU; 1 C =
= 6.241 x 1018 e; 1 A = 1 C / 1 s,
where "e" is the elementary charge of the electron.
Under the assumptions of using a coherent beam with resonating photons (today produced from synchrotrons of a few meters in diameter) hitting a sufficient mass of Mo(100, 42, 0) suitable to produce 1020 stimulated nuclear transmutations (102) per our, we have the following (see the figure):
Hadronic production of heat: 2 x 1020 MeV/h = 3 x 104 BTU/h,
Figure 7.37. Hadronic production of electricity: 2 x 1020 e/h = 200 C/h = 55 mA.
Needless to say, the above is merely an illustrative example, with numerous possibilities for improvements, such as the production of much bigger heat via the selection of a heavier hadronic fuel, the increase of the efficiency by adding triggers, etc.
7.4F. Tsagas experiment on the Stimulated Neutron Decay
The experimental verification of stimulated nuclear transmutation (7.53) was initiated by N. Tsagas and his group at the Nuclear Engineering Department of the University of Thrace, Xanthi, Greece, with preliminary, yet positive results presented in the paper
N. F. Tsagas, A. Mystakidis, G. Bakos, and L. Seftelis, Hadronic J. 19, 87 (1996).
The test was conducted quite simply by using a disk of the radioactive isotope Eu(152, 63, 3) as the source of resonating photons placed next to a disk of natural Molybdenum as target while measuring: the background: without any source; the emission with the Europa source alone; and the emission with the joint disks of Europa and natural Molybdenum.
Electrons originating from the Compton scattering of photons with peripheral atomic electrons can at most have 1 MeV energy, as well known. Therefore, the detection of electrons with energy over 2 MeV or more establishes their nuclear origin.
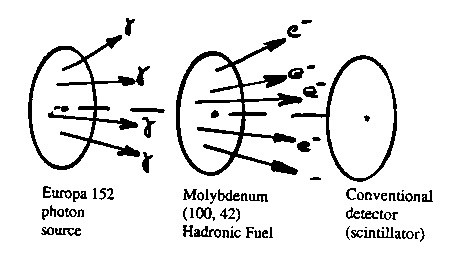
Figure 7.37.The set up of Tsagas experiment [44] on Santilli's stimulated decay of the neutron [43].
Since the Europa source does not emit electrons, and the Molybdenum is stable, the only possible origin of emitted electrons is due to the stimulated decay of neutrons inside the Molybdenum disk. As recalled earlier, the first reaction (6.2.236a) emits electrons with minimal energy of $2.8 ~MeV$, while the second reaction emits electrons with energy ranging from 2.22 MeV to 3.38 MeV.
It should be indicated that Tsagas's test has the following limitations [24]:
A) The tests used ordinary Molybdenum, that contains the isotope Mo(100, 42, 0) only in 0.6 %, while all the remaining isotopes of the Molybdenum cannot admit the stimulated decay here considered for various reasons.
B) The primary frequency emitted by the Europa isotope, 1.874 MeV, is not the resonating frequency that should instead be 1.294 MeV less the correction due to the nuclear binding energy, although Eu(152, 63, 3) does emit a number of additional photons, one of which has the energy of 0.148 MeV close to the subharmonic of the resonating energy.
C) The tests solely used detectors of the energy of the emitted particle, without additional detectors for the identification of their nature.
Under these conditions, the possibilities of achieving reaction (102) are rather limited. Yet Tsagas did indeed report the detection of emissions in the sole Eu-Mo coupling in excess of 1 ~ MeV, as shown in the figure below.
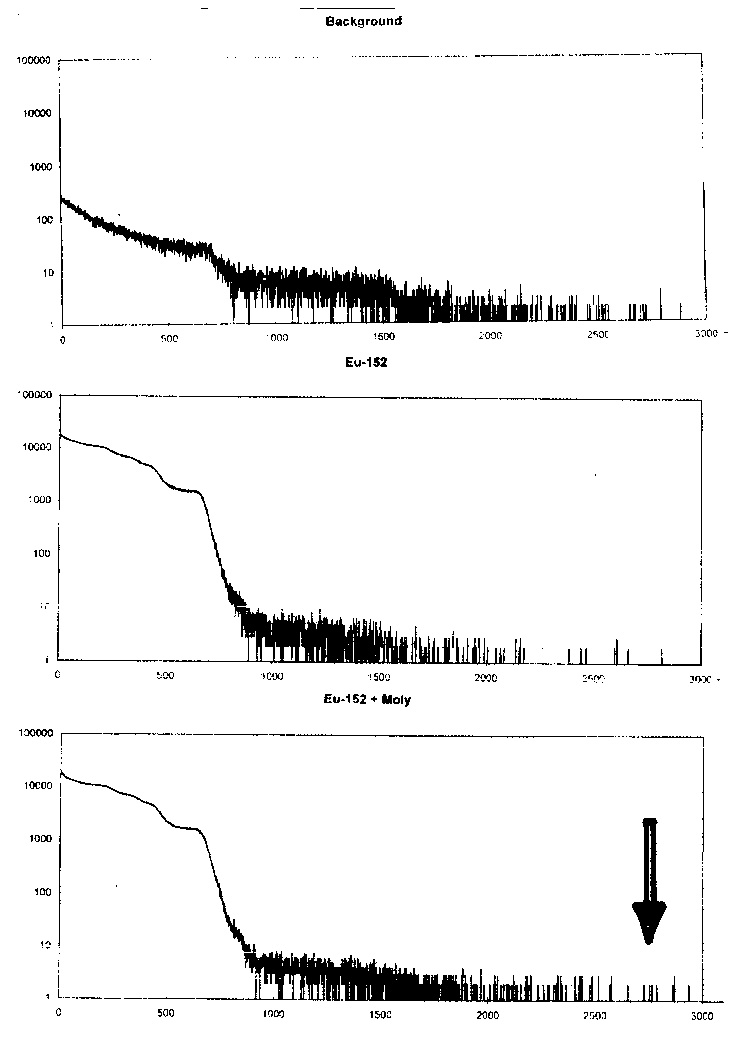
Figure 7.38. A view of the detection by Tsagas for the background, the Europa isotope alone, and the Europa-Molybdenum paid (below) showing the detection of emission over 1 MeV that can solely be of nuclear origin, thus confirming, although in a preliminary way, Santilli prediction [43].
n summary, far from being final, Tsagas tests remain the first experiment on Santilli's hadronic energy and, despite their limitations, they were indeed positive. It is regrettable, and in actuality hard to believe, that the nuclear physics community has ignored the finalization of Tsagas experiment for its confirmation or dismissal, despite its very moderate cost, while preferring much more expensive experiments that, however, are fully aligned with 20th century sciences.
7.4G. Recycling of radioactive nuclear waste via their stimulated decay
One of the most important implications of Santilli's studies on the structure, synthesis and stimulated decay of the neutron is their application to the recycling of highly radioactive nuclear waste via its stimulated decay as presented in the paper
R. M. Santilli, in Large Scale Collective Motion of Atomic Nuclei, G. Giardina et al., Editors, World Scientific (1997).
A scholar presentation is available in the book
J. Dunning-Davies, Exploding a Myth, Conventional Wisdom or Scientific Truth? Horwood (2007).
A review of the studies including and indication of the obstructions against their realization can be found in the website
W. Pound, "Recycling of nuclear waste"
http://www.nuclearwasterecycling.com
Needless to say, the expected solution is expected from a combination of methods, including Santilli's stimulated decay, but also including other approaches some of which have been patented. Most importantly, the equipment expected from these efforts is sufficiently small to be usable by the nuclear power plants themselves, thus avoiding the very dangerous and extremely expensive transportation of the waste to depositories for our descendants to recycle.
The implications are here far reaching because, on one side conventional nuclear power plants can become environmentally more acceptable while., on the other side, we can have the birth of a new multi-billion dollar industry.
Regrettably, the obstructions against the recycling of nuclear waste via its stimulated decay are beyond the imagination by most scientists. On one side, such as recycling would signal the termination of the dominance of quantum mechanics in nuclear physics.On the other side it would imply the termination of the ongoing one trillion dollars expenditures for the storage of the radioactive nuclear waste in the Yucca mountains. As a result, Santilli was forced to halt all his research in the field, and pledge never to resume it (as stated in His 2008 monographs) because of apparent life treats reported in the above quoted website.
The unreassuring information known to the authors is that, to our best knowledge at this writing (Fall 2010) no additional theoretical or experimental study in the recycling of radioactive waste via its stimulated decay has occurred since 1997.
7.5. THE STIMULATED DECAY OF RADIOACTIVE NUCLEAR WASTE
Under construction. Please visit the website
http://www.nuclearwasterecycling.com
7.6. CLEAN ENERGIES FROM THE NEW CLASS OF NUCLOIDS
,p>
To be uploaded. Visit in the meantime Section 6.4F of Chapter 6.
,p>
|